Artificial photosynthesis – the ability to convert sunlight, water, and carbon dioxide into fuel – has long been a green technology dream. Recent breakthroughs are bringing that dream closer to reality, promising a future where we can literally bottle sunshine. Scientists are mimicking the way plants use solar energy, but with a high-tech twist: instead of producing sugars, these systems generate energy-rich fuels like hydrogen, methane, or even liquid ethanol. The goal sounds simple but is deceptively daunting. “The biggest challenge many people don’t realize is that even nature has no solution for the amount of energy we use… Not even photosynthesis is that good,” says University of Chicago chemist Wenbin Lin. In other words, to meet our colossal energy needs sustainably, we must outshine nature’s own invention – and that’s a tall order. This exploration dives into the latest advances in artificial photosynthesis, how they compare to traditional solar panels and other renewables, and what it all means for our energy future.
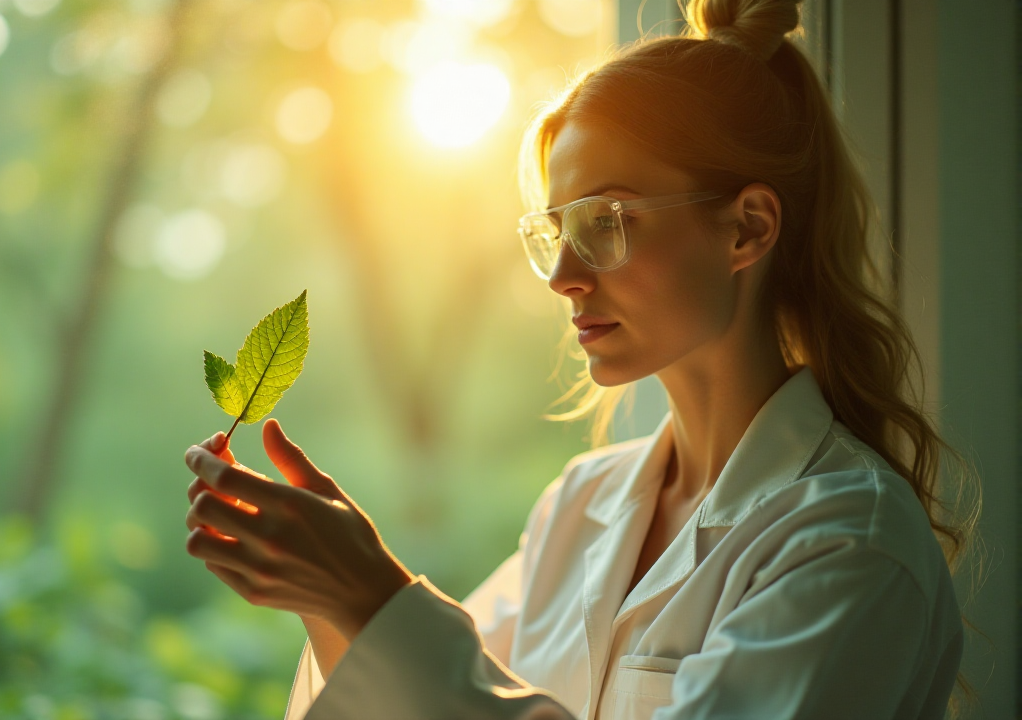
Table of Contents
ToggleLatest Breakthroughs: From Lab Prototypes to Solar Fuels
Recent years have seen rapid progress in artificial photosynthesis research, with scientists achieving feats once thought far-fetched. Here are some standout developments pushing the field forward:
- Sunlight to Ethylene – A Record-Setting U.S. Innovation: Researchers at the University of Michigan unveiled a device that turns carbon dioxide and water into ethylene (a key plastic feedstock) with unprecedented efficiency and durability. Ethylene is normally made from fossil fuels, but this solar-powered setup chains two CO2 molecules together into hydrocarbons. “The performance… is about five to six times better than what is typically reported for light-driven CO₂ reduction to ethylene,” said Professor Zetian Mi, who led the work. The system ran 116 hours without slowing down (some versions ran over 3,000 hours) and produced ethylene at a rate more than four times higher than previous systems. Such leaps in efficiency and longevity mark a key step toward viable “solar fuel” factories.
- Artificial “Leaf” Makes Liquid Fuel in One Step: In Cambridge, UK, scientists achieved a milestone by directly producing liquid fuels (ethanol and propanol) from sunlight, CO₂ and water. Until now, artificial leaves mostly yielded simple gases like hydrogen or carbon monoxide. “Shining sunlight on the artificial leaves and getting liquid fuel… is an amazing bit of chemistry,” said Dr. Motiar Rahaman, first author on the 2023 Nature Energy study. By engineering a special catalyst of copper and palladium, the team’s device skips the usual intermediate syngas step and outputs energy-dense liquid fuel that could be used in existing car engines. “Even though there’s still work to be done, we’ve shown that we can go beyond the simplest molecules and make things that are directly useful as we transition away from fossil fuels,” added Professor Erwin Reisner, the project lead. The catch? It’s only a proof-of-concept with modest efficiency so far. But it demonstrates that more complex, practical fuels can be made with nothing but sunlight as an energy source – a groundbreaking proof that artificial photosynthesis can create drop-in fuels.
- Mimicking Plant Power at Scale: A team led by renowned Japanese researcher Kazunari Domen built a 130-square-foot solar fuel reactor (about the size of a small bedroom) and tested it outdoors for three days. The array of photocatalytic cells, coated with a sunlight-absorbing semiconductor, produced methane from CO₂ and water – effectively making natural gas renewably. This large prototype, reported in 2023, withstood varied weather and showed that scaling up artificial photosynthesis devices is feasible. The materials (strontium titanate and simple aluminums) are inexpensive and easily scalable. However, as the researchers acknowledge, efficiency needs to improve before such devices become viable for large-scale power generation. Still, the proof-of-concept suggests future systems could be adapted to produce not only fuels but also chemical feedstocks for plastics. Notably, this project drew support from the European Union and a Japanese energy company, signaling global interest and industry involvement in solar fuel technology.
- 10× Productivity Boost with Innovative Catalysts: At the University of Chicago, chemists developed an artificial photosynthesis system that is ten times more productive than previous systems. By integrating novel materials called metal–organic frameworks (MOFs) with amino acids, the team drastically improved the reaction efficiency for converting CO₂ and water into fuels like methane. “This is a huge improvement on existing systems,” said Professor Wenbin Lin, “and we were able to lay out a very clear understanding of how this artificial system works at the molecular level”. Such molecular insight is crucial, as it helps scientists tweak and optimize each step of the process. The UChicago device optimizes both halves of the reaction – water splitting and CO₂ reduction – and achieved reaction rates that surprised even the researchers. “We will have to do better than nature, and that’s scary,” Lin quipped, noting that even plant photosynthesis isn’t efficient enough to meet human energy demands. The result is a new direction for artificial photosynthesis that bridges fundamental science and practical performance.
- Floating Fuel Factories: In a creative twist, Cambridge scientists also designed ultra-thin, flexible “leaves” that float on water and produce fuels from sunlight. Imagine rafts of solar fuel devices bobbing in a river or ocean, slurping up sunlight and CO₂ to output hydrogen or syngas. Outdoor tests on the River Cam showed these lightweight devices can generate fuel as efficiently as plant leaves floating on the water’s surface. “This is the first time that clean fuel has been generated on water,” the team reported, suggesting that future artificial leaves could operate in polluted waterways, ports, or at sea. By moving production to open water, they avoid competing with farmland or urban space. “If we can trim the materials down far enough that they’re light enough to float, then it opens up whole new ways that these artificial leaves could be used,” said Professor Reisner. The latest leaf prototypes use perovskite solar materials on flexible plastic, protected by micrometer-thin waterproof coatings. They’re “compatible with modern fabrication techniques, representing an early step towards the automation and up-scaling of solar fuel production,” according to Dr. Virgil Andrei, co-lead author. While still experimental, the vision is bold: “Solar farms have become popular for electricity… we envision similar farms for fuel synthesis,” says Andrei, floating on lakes, along canals, or offshore. It’s a glimpse of a future where fuel-producing sheets could cover reservoirs or coastlines, churning out green fuels for ships or industries and simultaneously reducing evaporation from water bodies.
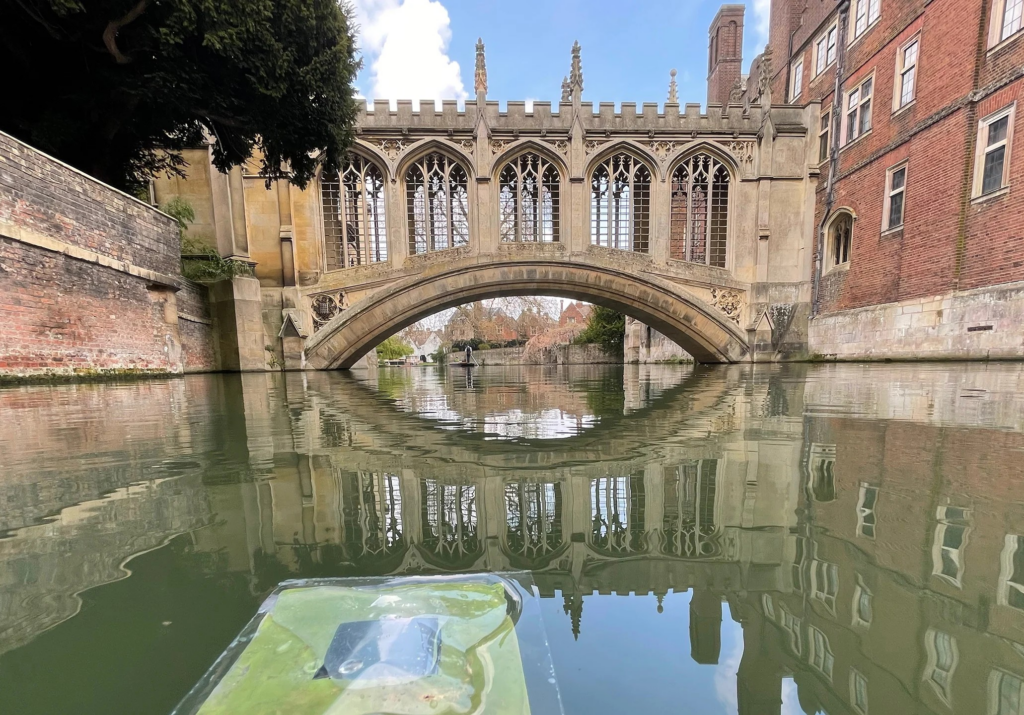
These examples – from American universities and national labs to international collaborations in Japan and Europe – underscore the momentum behind artificial photosynthesis. Scientists are attacking the problem from multiple angles: semiconductors and nanowires, molecular dyes, bio-inspired catalysts, and engineering tricks to make devices thin, robust, and scalable. The results are increasingly encouraging: higher efficiencies, new fuel products, longer-lasting systems, and larger demos.
Chasing Efficiency: How Do Artificial Leaves Stack Up?
One major yardstick for any solar technology is efficiency – how much of the sun’s energy it can convert into usable form. Natural photosynthesis in plants is surprisingly sluggish, converting only about 1% (at best a few percent) of sunlight into chemical energy (biomass). Traditional solar panels (photovoltaics), on the other hand, routinely convert ~20% of sunlight into electricity, with the best lab cells exceeding 40% under concentrated light. So, where does artificial photosynthesis stand in this race?
- Closing the Gap with Nature: Early artificial photosynthesis devices barely beat plants. But recent systems have leapfrogged natural photosynthesis in efficiency. As far back as 2017, a Berkeley Lab team integrated solar cells with custom catalysts to achieve solar-to-fuel efficiency over 5% for converting CO₂ into ethanol and ethylene– several times greater than what crops typically manage. “We did a little dance in the lab when we reached 5 percent,” recalled Berkeley scientist Joel Ager. That might sound low compared to a solar panel, but making chemical bonds (fuels) is a more complex task than making electrons flow. Reaching 5% was a “level of performance… people did not think was possible at this point,” said Dr. Frances Houle, JCAP’s science integration lead. It proved that artificial systems can rival and even surpass plants in turning sunlight and air into stored energy.
- Pushing into Double Digits: With relentless R&D, efficiencies have climbed further. Multi-junction approaches (tandem solar absorbers with optimized catalysts) have achieved 10–19% efficiency for solar fuels in lab settings. In fact, an international team including U.S. DOE’s Joint Center for Artificial Photosynthesis (JCAP) recently demonstrated a solar-driven fuel generator at 19% efficiency– a milestone putting it on par with commercial solar PV. (For context, a 19% solar-to-hydrogen efficiency means nearly one-fifth of the sunlight’s energy is stored in hydrogen fuel, a big jump from single-digit past results.) Such achievements typically involve expensive setups or ideal lab conditions, but they show what’s physically possible. The University of Chicago’s MOF-based system similarly boasted an order-of-magnitude jump in productivity, signaling that new materials and catalysts can unlock huge gains.
- Real-World Efficiency vs Theoretical Potential: It’s important to note that many breakthroughs reported are at prototype scale (square centimeters to a few square meters) and under controlled or simulated sunlight. Efficiency often drops when scaling up due to losses, imperfect light capture, or non-ideal weather. The 130-square-foot methane generator in Japan, for example, ran in real sunlight but acknowledged efficiency remains too low for commercial use. Many prototype “artificial leaves” today operate in the low single-digit percentages of efficiency when producing complex fuels. By contrast, off-the-shelf solar panels consistently deliver 15–20% efficiency in the field. This means that, at present, you’d need a much larger area of artificial photosynthesis devices to match the energy output of a solar farm.
- Beyond Efficiency – Selectivity and Stability: Another aspect of performance is what products are made and for how long. Nature’s leaf makes exactly what the plant needs (sugars), but early artificial systems often produced a jumble of chemicals or would corrode quickly. Here too there’s progress: catalysts are getting more selective, targeting desirable fuels (like the Michigan device specifically making ethylene or Cambridge’s leaf aiming for ethanol). Durability is improving with better materials and self-healing designs – e.g. that Michigan nanowire device ran over 100 hours with no degradation, and other teams are using protective coatings to prevent rust and catalyst “death”. So while efficiency grabs headlines, these practical advances in longevity and precision also drive the technology toward viability.
In summary, artificial photosynthesis has vaulted from fractions of a percent efficiency a decade ago to potentially double digits in the near future. It still generally lags behind standard solar panels in raw efficiency, but the gap is narrowing. More importantly, the form of the energy is different: solar panels give electricity (instant but hard to store), whereas artificial photosynthesis gives fuel (stored chemical energy). This distinction is crucial when comparing their value and use cases.
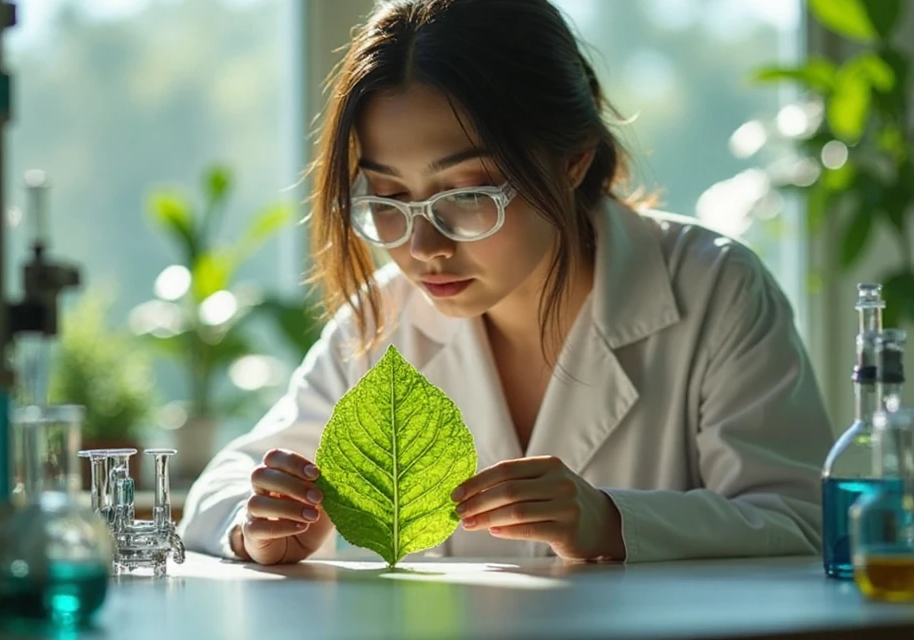
How Does Artificial Photosynthesis Compare to Other Renewables?
With solar panels, wind turbines, and batteries becoming commonplace, one might wonder: Why pursue artificial photosynthesis at all? How do these sun-fueled chemical factories stack up against other green technologies in efficiency, scalability, and economics?
Solar Panels vs. Artificial Photosynthesis
Traditional solar PV is a mature technology – prices have plunged by ~85% in the last decade, and global installed capacity is measured in hundreds of gigawatts. Solar panels directly generate electricity with high efficiency and reliability, which makes them extremely scalable for power generation. However, storing that energy long-term or converting it for use in vehicles, industry, or nights and winters requires additional steps (batteries, grid infrastructure, or making hydrogen via electrolyzers).
Artificial photosynthesis, by contrast, directly produces a storable fuel. This could be hydrogen gas to power fuel cells, methane or syngas to feed into existing pipelines, or liquid fuels for cars and jets. In essence, it’s solar energy storage built into the process. A conventional solar farm plus an electrolyzer to make hydrogen is effectively doing the same job as an artificial photosynthesis system – just as two separate pieces of equipment. The integrated approach could, in theory, simplify the system and lower costs if materials are cheap.
- Efficiency: Today’s best solar panels still outshine artificial leaves in converting sunlight to immediate energy (20% vs single-digits percent for many solar-fuel devices). But when you consider the full chain – solar panel to electricity to electrolyzer to fuel – the overall efficiency drops. For example, a solar panel (20%) feeding a water electrolyzer (~70% efficient) nets about 14% sunlight-to-hydrogen efficiency. Advanced artificial photosynthesis prototypes are approaching this range in a single device. In short, solar-fuel systems are catching up to the combined performance of PV+electrolysis, at least at lab scale. The ultimate vision is to beat that by using tailored chemistry that can exceed the sum of its parts – essentially doing better than off-the-shelf solar cells tied to off-the-shelf chemistry. Achieving ~20% fuel efficiency in one step would put artificial photosynthesis on equal footing with the best renewable setups, a target researchers are actively chasing.
- Scalability: Solar panels benefit from massive economies of scale and a huge manufacturing base. Gigafactories pump out silicon wafers by the ton. Artificial photosynthesis devices are where solar PV was decades ago – mostly handmade in labs. Scaling them up is a major challenge. Some paths look inherently scalable (like photocatalytic sheets or panels that could be made similar to how solar panels are made). For instance, the Domen team’s 130-ft² methane panel used abundant materials and simple coating techniques to cover a large area. Likewise, Cambridge’s ultrathin floating leaves were made using techniques from the electronics industry (thin-film deposition on flexible foils), hinting they could be produced in roll-to-roll processes like a plastic film. Researchers are keenly aware of this: “This study demonstrates that artificial leaves are compatible with modern fabrication techniques… an early step towards automation and up-scaling,” Dr. Andrei noted. Still, no one has yet built an “artificial photosynthesis farm” at anywhere near the scale of today’s solar farms. The coming years will tell if these devices can be mass-produced cheaply or if they remain bespoke apparatuses.
- Economic Viability: This is the elephant in the room. Right now, solar panels paired with batteries or grid power are usually cheaper than fancy new tech. A kilowatt-hour of solar electricity is often the cheapest energy on Earth today. By contrast, solar fuels are still expensive per unit energy because the tech is new and unoptimized. A sobering lesson came in 2011: Harvard’s Daniel Nocera built a famous “artificial leaf” that made hydrogen from sunlight using relatively cheap materials, and he even founded a company to commercialize it. But he soon “realising that the technique was still unlikely to compete with cheaper hydrogen produced by steam reforming of fossil fuels” and shelved the plan. Fossil fuel processes had a century head start, and even now, without a price on carbon, it’s hard for a solar chemical plant to compete with simply digging up natural gas. Policy could change this equation: if governments set stricter carbon targets or provide incentives for green hydrogen and fuels (as we’re beginning to see), then the economic balance shifts. Technologies like artificial photosynthesis stand to benefit from carbon credits, clean fuel standards, or investments via climate-focused funds. In the U.S., programs like the Department of Energy’s JCAP and ARPA-E funding are public bets on the long-term payoff of solar fuels. Europe and Japan are likewise heavily funding artificial photosynthesis as part of broader climate innovation strategies.
Beyond Solar: Wind, Biofuels, and Others
How about other renewables? Wind energy is a powerhouse for electricity generation, often complementary to solar PV. But like solar, wind just gives electricity – great for powering homes or EVs, but not directly yielding liquid fuels or industrial feedstocks. You’d still need an intermediate step (e.g., use wind power for electrolysis to make hydrogen or to drive CO₂ conversion in a separate reactor). Artificial photosynthesis could theoretically use any light source, but it’s optimized for sunlight – so it’s not competing with wind so much as offering a different service: renewable fuel production.
Biofuels do produce liquid fuels, but rely on natural photosynthesis (growing corn, sugarcane, algae, etc.). This approach, while currently the main source of renewable fuels, is constrained by land and often controversial. Huge swaths of farmland are used to grow bioethanol feedstock – nearly 45% of U.S. corn is used for ethanol– raising food-vs-fuel dilemmas. “Biofuels like ethanol are a controversial technology, not least because they take up agricultural land that could be used to grow food instead,” notes Prof. Reisner. Artificial photosynthesis, by operating on rooftops, deserts, or floating in water, sidesteps the land use issue. It also can be more efficient. Traditional biofuel pathways convert only a fraction of sunlight into crop growth (again ~1%), whereas an artificial system might use that same sunlight on the same area to produce significantly more fuel energy without the agricultural overhead. In essence, solar fuels promise more energy per acre than biofuels, with no need for fertile soil or fresh water for irrigation – a compelling advantage if realized.
Hydrogen economy technologies overlap with artificial photosynthesis too. Electrolyzers splitting water are already scaling up to provide green hydrogen for industry. These are powered by renewables like solar and wind. In comparison, a photocatalytic water-splitting panel (a direct artificial photosynthesis approach to hydrogen) could be simpler (no moving parts, no electrical grid connection) and perhaps cheaper in the long run. But current electrolyzers are fairly efficient and benefiting from industrial scaling. So, artificial water-splitting devices will need to prove they can beat or at least match the cost per kilogram of hydrogen. Some experts imagine hybrid setups: for example, “solar farms for fuel” where large artificial photosynthesis reactors float on reservoirs, complementing solar PV farms on land. The ultimate energy system might use both: traditional renewables for electricity and artificial photosynthesis for fuel and chemicals, each optimized for what they do best.
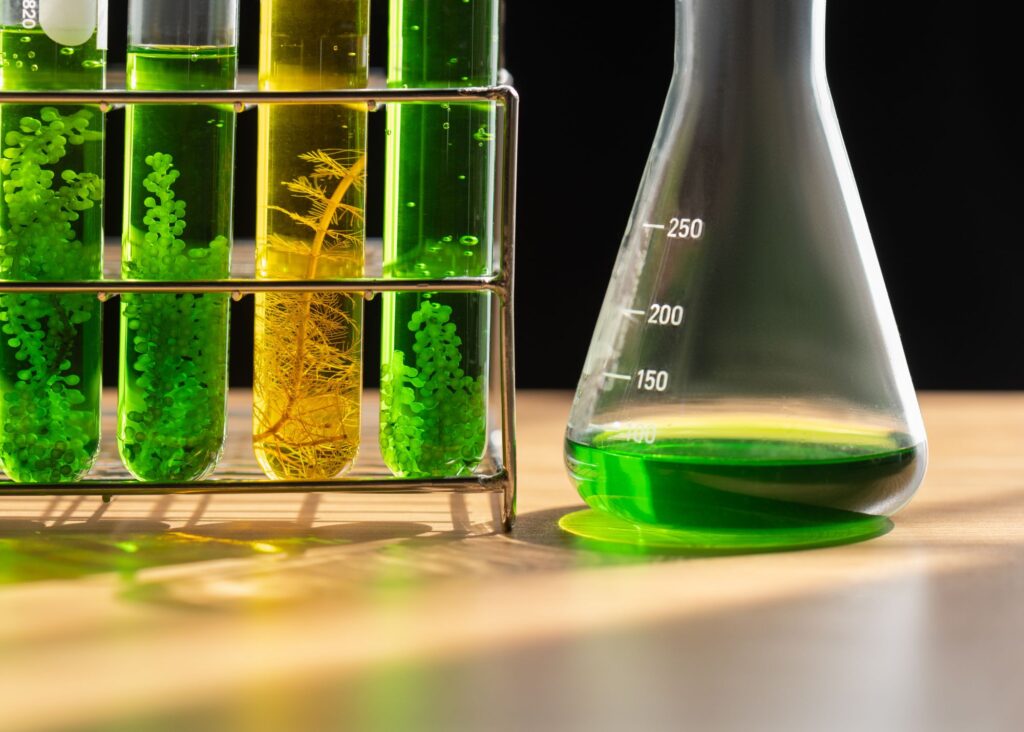
Applications of Artificial Photosynthesis: Fuels, Chemicals, and Industry Impact
What would widespread artificial photosynthesis enable? The potential applications extend across the energy and industrial landscape:
- Carbon-Neutral Fuels: The most obvious use is producing clean fuels for transportation – gasoline substitutes, diesel for trucks, jet fuel for airplanes, and hydrogen for fuel cell vehicles – all from sunlight and captured CO₂. For example, the Cambridge artificial leaf that makes ethanol/propanol directly could one day fill gas tanks with carbon-neutral ethanol (imagine truly “solar gasoline” that, when burned, releases CO₂ that came from air originally, closing the carbon loop). Likewise, methane made via artificial photosynthesis can be used in power plants or heating with no net CO₂ added to the atmosphere. These fuels would utilize existing infrastructure (pipelines, refineries, engines) but without the fossil carbon. This appeals greatly to sectors hard to electrify, like aviation and shipping, where high-energy liquid fuels are needed. Synthetic fuels made with renewable energy (sometimes called “e-fuels”) are already of interest; artificial photosynthesis could provide a one-step, potentially more efficient route to them.
- Green Hydrogen and Ammonia: Hydrogen is a cornerstone for decarbonizing industries (used in fuel cells, steel production, and refining) and ammonia is vital for fertilizers and could be a future shipping fuel. Today, both are made mostly from natural gas with heavy emissions. Artificial photosynthesis offers a way to produce hydrogen via water splitting using sunlight directly. In fact, some systems being developed target making ammonia from sunlight, water, and air (nitrogen) – essentially doing a solar-powered Haber-Bosch process. There have been experimental “bionic leaf” setups (like those by Daniel Nocera’s team) coupling microbes to solar catalysts to make ammonia or even complex molecules like biodegradable plastic precursors. While these hybrid bio-solar systems are a twist on artificial photosynthesis, they illustrate the breadth: everything from hydrogen gas to specialty chemicals could, in theory, be synthesized with solar energy.
- Chemical Feedstocks and Plastics: Beyond fuels for energy, artificial photosynthesis can produce the building blocks for chemicals and plastics. The University of Michigan’s ethylene device is a prime example – ethylene is used to make polyethylene (common plastic) and other products. Instead of drilling oil to crack into ethylene, a future plastics plant could pull CO₂ from an industrial exhaust or the air and, with sunlight, create ethylene to feed its reactors. “One direct application of the system would be to harvest carbon dioxide that would otherwise be vented… for making plastics,” the Michigan team noted. This not only reduces fossil resource demand but helps recycle CO₂. Similarly, CO₂-to-CO (carbon monoxide) via artificial photosynthesis can feed into Fischer-Tropsch reactors to make longer hydrocarbons, or be used to synthesize methanol, which is a feedstock for many chemicals. The versatility of outputs (hydrogen, CO, syngas, alcohols, methane, etc.) means solar-fuel devices could become a new kind of refinery – one that runs on sunlight and cleans the atmosphere as it produces commodities.
- Decentralized Energy Solutions: Because artificial photosynthesis devices don’t necessarily need electrical infrastructure, one intriguing application is in remote or off-grid areas. Picture a village in a sunny developing region that lacks reliable electricity: a simple photocatalytic reactor could generate cooking gas (hydrogen or methane) from a tub of water and sunlight, replacing wood burning or expensive propane deliveries. Or consider emergency fuel production – portable solar fuel generators could provide fuel for relief operations after disasters, using only sunlight and any available water. While these scenarios are still speculative, the inherent simplicity of some artificial photosynthesis designs (basically sun + water + catalyst, no external grid needed) could make them suitable for distributed use. Researchers have even floated the idea of solar fuel devices that double as shade or awnings, producing fuel on-site at farms or homes. The Cambridge group’s vision of rollable, lightweight fuel-producing sheets that “you could roll up… and put almost anywhere, in almost any country” speaks to this potential.
Overall, artificial photosynthesis could infiltrate many sectors that solar panels alone can’t touch. It blurs the line between energy and manufacturing – turning power plants and chemical plants into one. With that, however, come significant challenges in scaling up from a lab wonder to an industrial workhorse.
Challenges on the Road to Scale
For all the excitement, significant hurdles remain before we’re pumping artificial gasoline or rolling out artificial leaf farms. Experts are quick to temper optimism with practical realities:
- Scaling Up Reaction Systems: A small demo making a few milliliters of fuel is very different from a full-scale plant producing barrels. “Where we are now, it would need to scale up by many orders of magnitude to make a sufficient amount of methane for our consumption,” Prof. Wenbin Lin conceded frankly. Many current systems rely on rare materials or intricate nanostructures that are fine at lab scale but impractical in bulk. The path from an efficient catalyst in a beaker to a factory the size of a refinery is non-trivial. Engineering challenges include how to efficiently collect and separate the products (gases bubbling out of thousands of photocatalytic panels, for instance), how to maintain performance over months and years outdoors, and how to handle dynamic conditions (night/day cycles, weather swings) while still delivering steady output. These are the kinds of problems that chemical engineers and industry pilots will need to solve.
- Materials and Durability: Many ideal semiconductor materials for capturing sunlight (like silicon or perovskites) hate the reactions conditions – they might corrode in water or in contact with harsh reaction intermediates. A big focus is finding catalysts that don’t degrade or developing protective coatings. As Yale’s Shu Hu explained about solar water splitters: “Many materials corrode… especially under the extreme pH… It’s hard to say which approach will win, but so far protective coating schemes have won out.” Researchers are essentially trying to make these systems self-healing – one clever aspect of the Michigan ethylene device was that its gallium nitride semiconductor actually used the oxygen byproduct to heal its surface, maintaining performance. Another challenge is the catalyst selectivity – preventing side reactions that form gunk or unwanted products that could foul the system. Each component (light absorber, catalyst, membrane, etc.) must be robust and harmonize with the others. This fine-tuning is an ongoing battle.
- Efficiency vs. Cost Trade-offs: Pushing efficiency often means using exotic multi-layered solar cells, precious metal catalysts, or ultra-pure conditions – which drives up cost. On the other hand, using cheap materials (iron, nickel, simple metal oxides) might be very cost-effective but with lower efficiency. The sweet spot for commercial success will lie somewhere in between. For example, the 12% efficient artificial leaf by NREL in 1998 used rare metals and was impressive but not economical. In contrast, newer approaches aim for “good enough” efficiency with earth-abundant materials. It’s telling that many teams, like Reisner’s, emphasize using low-cost materials even if current efficiency is modest– because in the real world, cost per unit of fuel will matter more than record efficiencies. The involvement of companies (like the oil & gas company INPEX in Domen’s project or the startup NX Fuels licensing the Michigan tech) indicates that scaling and cost are on the minds of investors. They will be looking for approaches that can compete economically, not just scientifically.
- Infrastructure and Safety: If we do have artificial photosynthesis plants or devices all around, we need to handle the fuels produced. Hydrogen, for instance, is a tiny molecule that can leak and needs special storage (and has different safety concerns than gasoline). Methanol or other liquid fuels made from CO₂ are toxic and need careful handling, although they fit into existing fuel logistics better. Integrating these new production sources into the existing energy infrastructure (pipelines, storage tanks, fueling stations) requires planning. There’s also the matter of sourcing CO₂ for feedstock: artificial photosynthesis doesn’t magic fuel from nothing – the carbon has to come from somewhere. In a closed loop, it would ideally come from captured CO₂ (either direct air capture or industrial emissions). That means coupling these devices with CO₂ capture technology, which is another layer of complexity (and cost). On the flip side, if that puzzle is solved, it means we could actively draw down CO₂ while producing fuel – a double climate win.
- Policy Support and Market Creation: As mentioned, without a price on carbon or other incentives, fossil-derived fuels will undercut solar fuels for a while. Policy changes could rapidly make artificial photosynthesis economically attractive. For instance, if sustainable aviation fuel is mandated to comprise X% of airline fuel by a certain date, there will be a huge market for non-fossil jet fuel – which solar fuel makers could tap. Governments might subsidize solar fuel pilot plants or guarantee purchases (similar to how some countries spurred early solar PV adoption). Investment from climate funds or industrial giants could also accelerate progress. A historical parallel is how solar PV itself was once extremely costly – only through decades of R&D investment and initial subsidies did it become cheap. A similar story could play out for artificial photosynthesis: in the short term, it likely needs support; in the long term, it could become a game-changer for a fossil-free economy.
A Thought-Provoking Future
Artificial photosynthesis sits at the intersection of climate science, renewable energy, and even philosophy. It compels us to rethink what fuel is – not a relic of prehistoric sunshine (fossil oil and coal), but something we can manufacture fresh, on demand, from current sunlight. Imagine a future where oil rigs are replaced by “sun rigs” – fields of solar fuel generators – and tanker ships carry fuels that were made from thin air and sunshine. It’s a future where the line between energy and environment blurs: our power plants would consume carbon dioxide instead of emitting it, essentially using the atmosphere as the raw material for energy.
Experts often emphasize that artificial photosynthesis is not about replacing solar panels or wind farms, but complementing them. Each renewable solution has its niche. “Without natural photosynthesis, we would not be here… But it will never be efficient enough to supply fuel for us to drive cars; so, we will need something else,” as Wenbin Lin put it bluntly. The “something else” could very well be artificial photosynthesis filling in the gaps that electrons and batteries can’t fill – powering planes, trucks, and industrial processes, and providing seasonal energy storage.
There’s also an almost poetic aspect to it: after over a century of digging up carbon and releasing ancient CO₂, humanity is working on a way to put that genie back in the bottle using sunlight. It is a full-circle moment – leveraging the same fundamental process that gave us fossil fuels (photosynthesis, albeit over ages) but in a sped-up, high-tech form. As one Berkeley Lab researcher said, “As rising atmospheric CO₂ levels change Earth’s climate, the need to develop sustainable sources of power has become increasingly urgent. Our work here shows we have a plausible path to making fuels directly from sunlight.” It’s a path where chemistry, nanotech and climate science converge.
Public opinion and excitement around these breakthroughs are growing as well. Media outlets have dubbed some prototypes “artificial leaves” in a nod to their inspiration. Floating fuel devices in Cambridge even caught attention as “floating factories of artificial leaves” that could one day fuel jets and ships. Such imagery captures the imagination: rivers and seas covered with thin green sheets generating clean fuel, perhaps with ducks curiously swimming by (as they did in Cambridge’s tests). While it’s crucial to stay grounded – these are early days – it’s hard not to be intrigued by the possibilities.
The coming decade will be critical for artificial photosynthesis. Will it remain mostly in research labs, inching forward in efficiency tables? Or will we see the first pilot plants and commercial units proving their worth? Signs of progress are encouraging: patents are being filed and startups formed, governments are funding “moonshot” programs, and interdisciplinary collaborations (chemists, engineers, biologists working together) are accelerating innovation. Each breakthrough – a more durable catalyst here, a more efficient light absorber there – builds the toolkit needed to design viable systems.
From an editorial perspective, it’s clear that artificial photosynthesis has shifted from pure science fiction to a plausible part of our future energy mix. It invites a broader conversation about investment and patience. We tolerated solar panels being expensive and inefficient in their infancy because their promise was huge – today that bet has paid off with some of the cheapest power in history. Now, a similar bet is being placed on these sunlight-to-fuel technologies. They tackle some of the hardest remaining decarbonization problems and, if successful, could fundamentally change how we power our world.
In conclusion, artificial photosynthesis is a field to watch as we strive to solve the energy puzzle in a sustainable way. It provokes us to imagine a world where every ray of sunshine can not only keep the lights on, but also fill our gas tanks and feed our industries – without warming the planet. The challenges are real, but so is the ingenuity being unleashed to overcome them. “It’s important to show that we can go beyond the simplest molecules and make things that are directly useful,” says Reisner– a sentiment at the heart of this pursuit. We are, in a sense, engineering a second photosynthetic revolution, one that could power human civilization in harmony with the environment. Achieving that will require science, yes, but also sustained public interest, smart policy, and a willingness to invest in the long term. If we succeed, the payoff is nothing less than the ability to fuel our global economy on sunshine and air – an enticing and profoundly hopeful prospect.