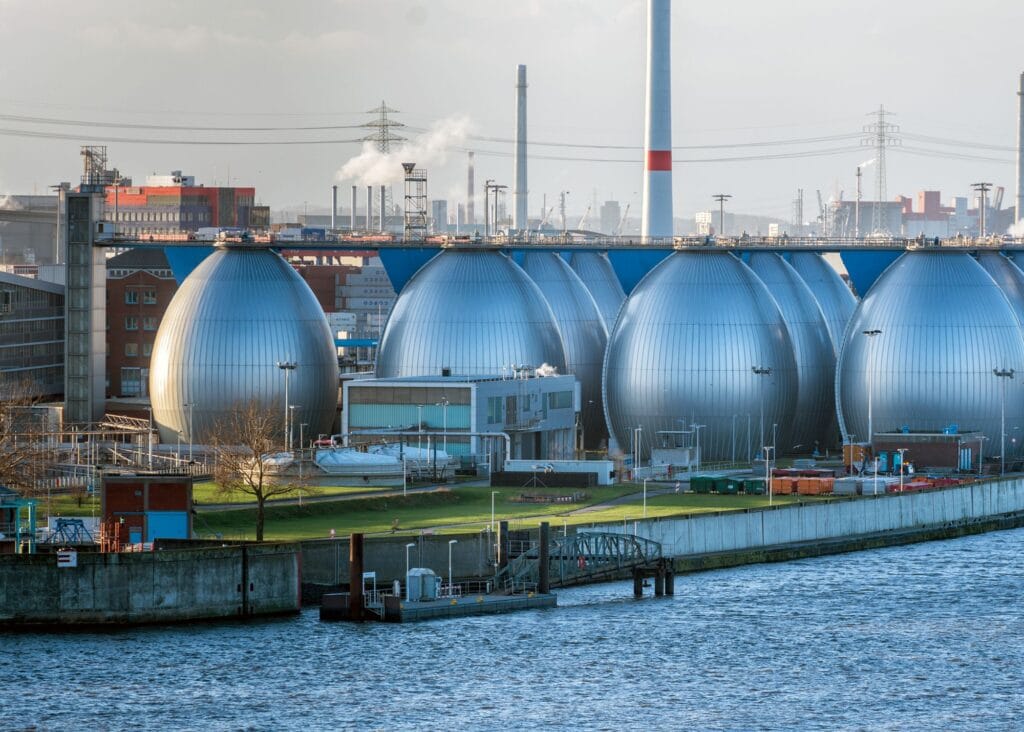
Table of Contents
ToggleA New Hope for Water-Scarce Regions
On May 12, 2025, at the Expo 2025 in Osaka, an Emirati startup named Manhat made headlines by winning a prestigious Best Practices Award. Manhat’s innovation – a passive, solar-driven floating desalination system – promises to produce fresh water from the sea without any external energy or carbon emissions. For policymakers and climate-focused investors, this development couldn’t be timelier. In an era of megadroughts and mounting water scarcity, the idea of generating fresh water “without electricity” has enormous appeal. But how significant is this technology really? Can a cluster of floating, zero-energy desalination units compete with – or complement – the giant land-based desalination plants that have so far dominated attempts to drought-proof our water supplies?
This editorial takes a hard look at Manhat’s passive desalination approach versus conventional reverse osmosis (RO) desalination. We will compare their economics, scalability, and environmental footprint, drawing on data from real projects (like California’s now-shelved Poseidon RO plant) and explore how such technology could be applied in U.S. coastal states. Along the way, we’ll examine regulatory hurdles – from state coastal commissions to federal ocean agencies – and consider whether policy shifts could accelerate adoption of novel offshore water tech. The goal is a clear-eyed, data-driven analysis of whether Manhat’s floating stills are a curiosity or a catalyst for the future of water.
The High Cost of Conventional Desalination
Large seawater RO desalination plants have earned a reputation as mega-infrastructure projects – expensive, energy-intensive, and often contentious. A prime example was the proposed Poseidon Water plant in Huntington Beach, California, which sought to produce ~50 million gallons per day (190,000 m³/day) of drinking water. The Poseidon project’s price tag was about $1.4 billion in capital cost, and critics warned it would drive up local water bills by over $100 million per year. In fact, the water from Poseidon would have cost nearly four times the price of existing supplies in Orange County. This mirrors the experience at California’s Carlsbad desalination plant (Poseidon’s earlier project near San Diego), which cost around $1 billion to build and now sells water at roughly $2,750 per acre-foot. For comparison, imported surface water in Southern California costs only about $855 per acre-foot. In other words, desalinated water can be 3 to 4 times more expensive than traditional sources – a gap that often ends up on ratepayers’ bills or requires government subsidies.
Operating costs are a big reason conventional desal is so pricey. Reverse osmosis works by forcing seawater through microscopic membranes at high pressure, which guzzles electricity. A typical seawater RO plant consumes on the order of 3–6 kWh of electricity per cubic meter of water produced. For a facility like Carlsbad (56,000 acre-feet per year, or ~69 million m³/year), that translates to hundreds of gigawatt-hours of power annually. Indeed, energy is such a major factor that when energy prices rise, the cost of desalinated water rises in tandem. Over 20 years, simply keeping Tampa Bay’s smaller 25 MGD RO plant running will cost over $500 million in operating funds. And despite modern efficiency improvements (energy recovery devices, optimized membranes), RO desalination still requires a steady supply of power – usually from the grid, which in many regions means fossil fuels. Globally, existing desalination plants emit around 76 million tons of CO₂ per year, and that could triple to ~218 million tons by 2040 as more plants come online. For climate-conscious investors, the carbon footprint of traditional desal is a serious black mark against it.
Then there are the environmental externalities. A large RO plant typically sucks in enormous volumes of seawater both for product water and to dilute the byproducts. Poseidon’s proposal, for instance, would have drawn in some 100 million gallons of seawater per day, using it to produce 50 million gallons of fresh water while the other half became concentrated brine. That brine – roughly twice the salinity of normal seawater – must be disposed of carefully to avoid killing marine life. Even with diffusers to mix it, the outflow from Carlsbad raises salinity in the vicinity by about 20% above ambient. “Most desalination plants discharge water with about 50% extra salt, which can lead to dead spots in the ocean” if not adequately dispersed. Environmental reviewers in California warned that Poseidon’s brine discharge and intake would “kill billions of tiny marine organisms” at the base of the food chain along the coast. In addition, conventional plants use chemicals (antifouling agents, chlorine, anti-scalants) in pre-treatment, and these can produce toxic by-products that end up in the waste stream. All told, a big RO desal plant can significantly alter the local marine environment, which is why permitting one is so difficult. In May 2022, after more than 20 years of debate, the California Coastal Commission unanimously rejected the Poseidon Huntington Beach project – despite a severe ongoing drought – citing the damage to marine ecosystems and the high cost to consumers.
Beyond environmental permits, these projects face logistical and timeline challenges. Carlsbad’s desalination plant took 14 years of planning and permitting before it finally opened in 2015. By then, initial cost estimates had nearly tripled. Supply chain complexity is partly to blame: building an RO plant requires specialized pumps, energy recovery turbines, high-grade pressure vessels, and miles of pipeline. There are membrane modules, typically imported or produced by a handful of manufacturers, that must be replaced every few years. Highly skilled engineers and contractors are needed to assemble and maintain the plant, and even after commissioning, unexpected issues can crop up (for example, Carlsbad has had to invest tens of millions more in new intake screens and antifouling measures to meet updated environmental requirements). All of this adds layers of cost and risk. In short, big desal plants are complex mega-projects with high CAPEX, significant OPEX, and multi-year lead times – factors that have limited their appeal as a quick solution to water crises.
Manhat’s Passive Floating Desalination – How It Works
In stark contrast to the industrial complexity of RO plants, Manhat’s desalination device is deceptively simple. The concept is essentially a high-tech take on the age-old “solar still.” Each unit is a floating structure, akin to a mini-greenhouse, that sits on the ocean surface and harnesses solar heat to evaporate seawater. As the sun warms the water under the device, pure water vapor rises and then condenses on the inside of the transparent enclosure. The condensed fresh water drains into a collection reservoir, while the salt is left behind in the sea. There are no high-pressure pumps, no external power supply, and no brine outfall pipes – nature does the heavy lifting. Dr. Saeed Alhassan, Manhat’s founder and CTO, describes it as “trapping the evaporated water mimicking the natural water cycle with zero carbon footprint and zero brine rejection”. In other words, it’s a man-made imitation of what the sun does across oceans every day, only on a smaller, controlled scale and with the ability to capture the evaporated freshwater before it forms clouds.
Crucially, Manhat’s design leaves the salt in the ocean without concentrating it into a harmful plume. Because the device is open to the surrounding sea (sealed on top but open at the bottom to the water body), any increase in salinity beneath the unit simply diffuses into the broader ocean. No salt ever accumulates to clog the device – the designers point out that “no matter how hard you try to evaporate the water, there won’t be any salt to accumulate” on an open water surface. This means zero brine discharge in the conventional sense; there is no point-source release of hypersaline water, just a very slight local increase in salinity that is continually dissipated by ocean currents. In effect, the ocean itself is the “brine pond,” handling salt disposal gradually and benignly, much as it does in nature. This tackles one of the thorniest environmental problems of desalination by design, rather than bolting on energy-intensive diffusers or blending systems as RO plants must.
Another notable aspect is energy (or the lack thereof). Manhat’s units use only solar radiation – there are no pumps or electrical components onboard. This makes the system passive and zero-carbon in operation. Whereas a typical RO plant requires a dedicated power supply (often a fossil-fueled power plant or a substantial allocation of grid renewable energy), a field of Manhat stills would require no external electricity at all. The startup claims its method produces water “with zero electricity”, eliminating carbon emissions entirely from the desalination process. Of course, energy is used indirectly in manufacturing the devices, but their operational carbon footprint is essentially nil. For countries and companies committed to decarbonization, that is a game-changing difference. It’s worth noting that solar desalination isn’t new – solar stills are used in remote areas and survival situations – but those are usually small, enclosed basins. Manhat’s innovation was to make it floating and open to the sea, which vastly expands the potential surface area (oceans cover 70% of the planet) and avoids manual salt removal.
The current prototypes give a sense of scale: Manhat has tested floating units about 3 meters by 3 meters (approximately a 10 ft × 10 ft footprint) as well as smaller 0.5 m × 0.5 m versions. The 3×3 m device, roughly the size of a small raft, is a modular tile that can be reproduced and deployed in large numbers. Manhat’s team has indicated they will standardize the size as they move from prototype to commercial product. Each unit is basically a “water factory” for one, producing fresh water continuously as long as the sun shines and temperatures provide a condensation gradient. The water collected can be piped or siphoned off to storage, or even used in situ for irrigation if crops are being grown on a floating platform attached to the still. In fact, the startup’s initial focus is on pairing these devices with floating farms – essentially, barge-based agricultural plots – so that the fresh water is used immediately on-site to grow crops. By generating irrigation water at the point of use, these floating farms would bypass the need for pumping water from shore and eliminate evaporation losses from open irrigation canals. It’s a closed-loop sustainable concept: the sun and sea provide water, which enables local food production without tapping aquifers or rivers.
From a deployment perspective, Manhat’s units are meant to be “easily deployable” and adaptable to various environments. They can be anchored along coastlines, floated in calm bays, or even placed in large artificial water bodies like reservoirs or industrial evaporation ponds. Because each module is relatively small and has few moving parts, the manufacturing and supply chain may resemble that of a consumer product more than a power plant. The devices are made of materials like acrylic or other durable polymers, assembled into a pontoon-supported greenhouse. The company notes it sources components and assembles the product in the UAE, essentially buying “different pieces and putting them together” in Dubai. This implies that, with the right molds and materials, production could be scaled in factories, potentially driving down unit costs through mass manufacturing (similar to how solar panel costs plunged once large-scale factory production took off). No exotic membranes or turbines are needed – a significant contrast to RO’s complex supply chain. Manhat has already secured patents in 18 countries including the U.S., China, India, and across Europe, indicating a broad interest in protecting the IP as they eye global markets.
Economics: CAPEX and OPEX Head-to-Head
A critical question for investors is whether Manhat’s approach can compete economically with conventional desalination. On the surface, the value proposition is compelling: no energy cost, no chemical pre-treatment, and minimal maintenance, which should translate to very low operating expenses (OPEX). The main costs would be the capital cost of manufacturing and deploying the units (CAPEX) and any periodic cleaning or replacement of the units over time. In theory, if a floating still can be built cheaply enough and lasts many years, the levelized cost of water (much like levelized cost of energy in solar PV) could undercut big desal plants that have ongoing power and chemical bills.
Let’s break down the considerations:
- Capital Investment per Volume: Conventional large RO plants benefit from economies of scale (bigger plants spread fixed costs over more output), but they also require a huge upfront investment in one location. The Poseidon plant was $1.4 billion for 50 MGD, which is $28 million per MGD of capacity – or roughly $7,400 per cubic meter/day. By comparison, a small passive unit might produce on the order of a few tens of liters per day under strong sun. To match 1 MGD (~3,785 m³/day) using, say, a device that yields 50 liters/day, you’d need on the order of 75,000 units of that size. If each unit cost (hypothetically) a few hundred dollars, the capital to deploy those 75,000 units might be on the order of tens of millions – potentially in the same ballpark as a traditional plant’s cost per MGD. The unknown right now is the actual manufacturing cost per unit at scale. Early prototypes are hand-made and expensive; however, if these can be mass-produced via molding plastics or composites, costs could drop significantly. Manhat claims the invention is “less expensive than existing systems” since it doesn’t require high-pressure pumps or thermal heaters. This suggests that material and manufacturing costs, even including installation, are expected to be lower than the intensive civil works and machinery of RO. Economies of unit replication (mass production) might replace the conventional economies of scale (bigger plant size), meaning the cost curve could improve rapidly with volume. For investors, this hints at a modular, scalable capex model: one could start with a pilot array of modest cost, then add units incrementally as demand grows or as the concept proves out, rather than sink a billion dollars upfront hoping demand materializes.
- Operating Costs: Here the passive design shines. A traditional RO plant has ongoing costs for electricity, filter replacements, membrane replacements, chemicals (for anti-scaling, cleaning, chlorination), skilled labor, and the eventual refurbishments of pumps and motors. Manhat’s floating stills, by contrast, consume no fuel or power, use no chemicals, and have no membranes. The OPEX is near-zero aside from cleaning the transparent surfaces (to remove algae or mineral film) and occasional repairs. The harsh marine environment is an enemy to any equipment, so we should assume divers or maintenance crews will need to scrub biofouling off the units or clear any debris – but that maintenance is more akin to cleaning solar panels or boat hulls than maintaining a power plant. If the materials are robust (e.g. anti-UV, anti-corrosion plastic), each unit could last many years with minimal upkeep. In pure energy terms, zero kWh per m³ is unbeatable. This means cost stability: unlike RO, which is exposed to electricity price volatility (as seen when energy hikes pushed Carlsbad’s water to $2,750/acre-ft), passive desal costs wouldn’t swing with energy markets. That predictability is valuable for water utilities budgeting for the long term.
One way to illustrate the difference: the Tampa Bay RO plant produces 25 MGD and costs about $13 million per year in energy and operations (given a $500M cost over 20 years). A comparable output from passive units would spend almost $0 on energy. Even if the passive units required a larger workforce to manage deployment and harvesting water, labor costs for a somewhat distributed system might be offset by not needing highly specialized operators (no high-pressure pumps to monitor, etc.). In remote or developing areas, the ability to produce water without a power plant or grid connection also means no fuel logistics and no electricity infrastructure costs – a huge advantage where power is scarce or expensive.
- Water Cost per Acre-Foot: Ultimately, policymakers care about the cost per volume of delivered water. For RO, as noted, that can range from $600/acre-ft (some brackish desal) to $2,000-$3,000+/acre-ft for seawater desal. Passive desal doesn’t have a track record yet to pin down a price. However, consider that water scarcity itself is driving prices upward: during California’s recent drought, spot prices for water rights shot above $1,100 per acre-foot in mid-2022. In a prolonged drought, urban users might tolerate even higher costs for a guaranteed supply. If Manhat’s system can deliver water in the low four-figures per acre-foot, it could find a ready market wherever traditional infrastructure can’t keep up with demand. As manufacturing scales and if unit lifespans are long, that cost could drop further, potentially competing with imported water or recycled water costs common in arid regions.
In summary, Manhat’s floating desal system shifts the cost structure from ongoing expenses to upfront investment, much like solar PV did for electricity. This makes financing models like capital leasing or pay-per-volume service possible: for instance, a company could deploy and maintain a field of desal floats and charge a municipality per cubic meter of water delivered, pricing it below the local utility’s marginal cost of water. Because capacity can be added in modules, there’s also flexibility to right-size the deployment – avoiding overshooting demand and wasting capital (contrast with a huge desal plant that might run under capacity if demand forecasts are off). For investors, this modular approach can reduce risk: you can fund a pilot, validate performance and costs, then scale up in phases rather than one massive bet.
Can It Scale? From Niche to Municipal Supply
The most significant challenge facing passive desalination is scalability: can we produce not just thousands, but millions of cubic meters of water per day with this method if needed? It’s one thing to supply a coastal farm or a village; it’s another to supply Los Angeles or Phoenix. Conventional desal plants are centralized behemoths by design – Carlsbad supplies about 400,000 people on its own. To match that with small units would require an extensive network. Let’s grapple with the scale question:
- Footprint: RO plants concentrate water production in a small footprint on land (e.g., Poseidon’s plant would have sat on ~12 acres onshore). Manhat’s approach, in contrast, spreads the collectors over the sea surface. If one 9 m² unit (3×3 m) produces on the order of ~40–50 liters a day (an estimate based on typical solar still yields in hot climates), it would take on the order of tens of thousands of such units to produce even 1 MGD. A city-scale supply (50 MGD) could require a sea area of several square kilometers covered by devices. This is both a drawback and, potentially, an opportunity for clever integration. Oceans have space, but not all of it is usable – shipping lanes, fishing areas, marine protected zones, and aesthetic concerns limit where one could deploy a huge array of floats. However, a distributed network of smaller clusters could be placed in patches along a coast or offshore where they don’t interfere with other uses. It may resemble offshore wind farms, which occupy sections of the sea with spaced-out installations. In fact, a floating desal array might be co-located under a wind farm – the seas deemed appropriate for turbines (in terms of depth and exclusion zones) could also host desal units in the relatively calm waters between turbine pylons. Still, the sheer number of units needed for large output means the concept is likely better suited to decentralized applications or augmenting supply, rather than replacing a city’s entire water system overnight. We might envision hundreds of units serving a coastal town, or a few thousand units providing a new water source for a cluster of farms. For large metropolitan needs, multiple deployments along the coastline could each feed into the grid, improving resilience (distributed sources are harder to knock out all at once).
- Modularity and Phasing: The flip side of needing many units is the ability to phase expansion. A traditional plant produces zero water until the whole thing is built and commissioned; a floating system could start delivering water as soon as the first batch of devices is in the water and plumbed to a tank. New units can come online sequentially, which means scaling can track demand growth. For fast-growing regions or those uncertain about future water use, this is appealing. It also aligns with budget cycles – instead of one lump-sum expenditure, a utility could invest gradually, and investors could potentially see returns sooner from initial operating units while later ones are still being installed.
- Integration with Existing Systems: Passive desal units produce pure distilled water. For potable use, this water may need remineralization (as Dr. Alhassan noted, totally pure water lacks certain minerals and “we still have to add some salt” to meet drinking water standards). This is a minor post-treatment step, but it means the water likely needs to be collected and processed centrally before distribution if used for municipal supply. That implies piping networks or collection barges to gather water from many units. Engineering a piping network among floating stills is not trivial – one could imagine a flexible hose manifold linking hundreds of units to a central collection tank. Alternatively, each unit could store a day’s water and a crew on a boat could go around pumping out the fresh water, though that’s labor-intensive. More efficiently, a grid of units might feed via hoses to a floating storage platform or directly onshore via a pump. These logistical questions are solvable, but they add to the complexity as the system scales. It’s possible that an optimal solution is a hybrid system: use passive units to supply certain uses (like irrigation or industrial water that can be used right at the coast), which then frees up existing potable water supplies for cities. Or use passive desal in tandem with a smaller RO plant – the RO plant could handle base load and the passive units provide peaking supply during drought, etc.
- Durability and Reliability: Scaling also depends on how well the units survive real-world conditions. A floating farm of desal units must contend with storms, waves, salt spray, and biofouling. The Persian Gulf, where Manhat began, has relatively calm seas; the open Pacific or Atlantic can be far harsher. For instance, could these units survive a Gulf of Mexico hurricane or a California Pacific winter storm? They might need to be towed to sheltered waters or designed to submerge in extreme weather, or simply accept some losses and replace units periodically. Part of scaling is maintenance infrastructure – crews, boats, spare parts – spread over potentially large coastal areas. This is a different maintenance model than a single plant with onsite staff, but it might leverage existing marine services (e.g., companies that service buoys, offshore wind, or aquaculture equipment could service desal floats). Ensuring reliability and uptime of thousands of small units could be a challenge without a good monitoring system, so likely each unit would have to be simple enough that even if some fraction are down or lost, the rest continue without significant overall impact.
In essence, Manhat’s tech scales by replication rather than by amplification. It invites a paradigm shift: thinking of water infrastructure not as one giant faucet, but as a network of many small “raindrops” collecting water. This distributed model is quite new to water utilities, but not unheard of – consider how solar panels on thousands of roofs now contribute to the energy grid, supplementing centralized power plants. Water could see a similar diversification, especially in coastal arid regions where every drop counts.
Environmental Footprint: Green Credentials and Trade-offs
Manhat’s floating desalination system appears to deliver major environmental advantages over RO: no carbon emissions, no concentrated brine discharge, and no heavy chemical usage. These points address the core critiques of conventional desalination. However, a fair analysis should consider any new environmental impacts that a large-scale rollout of floating stills might introduce:
- Marine Ecosystem Impact: The absence of intake pipes means no marine organisms get impinged or entrained (sucked into a system) – a big win for fish eggs, larvae, and plankton that otherwise can be harmed by RO plant intakes. Likewise, without brine outfalls, there’s no sudden spike in salinity or discharge of dechlorination chemicals at a single point. Instead, any salinity increase is diffuse. However, covering sections of the ocean surface with floating structures does have an ecological effect: it shades the water below, reducing sunlight for photosynthetic marine life (like algae or seagrass) underneath. If thousands of units were tightly spaced, the light reduction could create a localized “artificial cloud” on the sea. This might be negligible if units are dispersed or if placed in deep open water where little grows anyway, but nearshore ecosystems could notice it. That said, the footprint of each unit is small, and they likely would be spaced out (not edge-to-edge like a solid mat) to allow some light and flow between them. Artificial reefs or perches could form on the underside of the floats – marine organisms may actually colonize them, as happens with any structure at sea. In some cases, that could be a benefit (creating new habitat) or a nuisance (fouling by barnacles, etc., which adds weight and maintenance needs). Overall, compared to the impact of large intake pipes and brine dumps, the ecological disturbance of passive units seems far gentler, especially if sited considerately.
- Emissions: Zero operational emissions are a huge plus. We can’t overstate this: as countries strive for net-zero, the 76 million tons of CO₂ from today’s desal plants is a glaring problem. Passive desal, being solar-driven, directly displaces what would otherwise likely be fossil-fueled electricity use. In a place like the Middle East, where desal is often powered by oil or gas, a shift to passive solar desal could cut both carbon and fuel costs (which is why UAE, where Manhat originated, cares deeply about this innovation). Even if floating stills don’t entirely replace RO, any volume of water they provide is water that didn’t require emissions to produce – an important factor for meeting climate targets or avoiding emissions pricing costs in the future. Manufacturing the units will have an emissions footprint (plastics, fabrication, transport), but so does manufacturing an RO plant’s components. We would need a life-cycle analysis to compare, but intuitively, producing simple hollow structures should be far less carbon-intensive than producing miles of spiral-wound polyamide RO membranes, high-grade stainless steel pumps, and concrete buildings.
- Resource Use and Supply Chain: Passive units likely use plastics or composites, and possibly glass or aluminum for some parts. Scaling up would mean large-scale production of these materials, which has its own environmental footprint (e.g., plastic production from petrochemicals). Ideally, materials could be chosen to be recyclable or long-lasting to minimize waste. On the positive side, eliminating the need for RO membranes (which have a finite life and become landfill) and chemicals (which require manufacturing and can spill or cause harm in transport) simplifies the supply chain. There’s also no need for backup power generators (and diesel for them) at these installations. Decommissioning a passive desal farm would be simpler too – essentially just pulling out the floats – whereas a desal plant leaves a large industrial site that might have contaminated filters or infrastructure to dismantle.
- Aesthetic and Social Factors: One reason the Coastal Commission bristled at Poseidon’s plant was community opposition – not only environmental, but also the notion of industrializing the coast. A floating desal array will have visibility issues too: locals might object to seeing a field of odd floating domes off their beach. This is somewhat analogous to the controversy over offshore wind farms and their sightlines. However, smaller desal devices sitting low on the water would be less visible than wind turbines. They might look like a cluster of small boats or buoys. If kept a few miles out or along less-trafficked stretches of coastline, most people might not notice them. Fishermen and boaters would need to know their locations (to avoid entanglement or collision), so they become a part of maritime spatial planning. Compared to a giant onshore facility with pipelines and power lines, the nimble and removable nature of floating units could be seen as less permanent and imposing. Nevertheless, community engagement would be necessary to avoid NIMBY-style pushback – an array of desal floats is still a new use of ocean space that people will want a say in.
In balance, the environmental footprint per gallon of water from passive desal appears far lighter than from RO. The technology was explicitly developed to address the very environmental critiques of desalination, and it largely succeeds in that aim. It turns the ocean and sun into both the input source and the energy driver, with minimal external interference. For regions like the Arabian Gulf, where decades of intensive RO desal have raised seawater salinity and temperature (due to discharge and power plant cooling) and even altered local marine life, a shift to passive systems could allow some recovery. For places that have shied away from desalination due to environmental fears – California being case in point – this technology might make desal politically palatable where it was not before. A commissioner at the Poseidon hearing said “the ocean is under attack… I cannot say in good conscience that this amount of damage is OK.” With passive desal, a regulator could find the environmental damage to be negligible or at least mitigatable to OK levels, changing the calculus in permitting decisions.
U.S. Applications: From California to Florida
The United States, despite having significant coastline and drought-prone regions, has been cautious in embracing seawater desalination. California, Arizona, Texas, and Florida each face water challenges where a solution like Manhat’s could play a role – albeit in different ways.
- California: The American West is emerging from a megadrought that a 2022 study deemed the worst in 1,200 years. California’s reservoirs and aquifers have been strained by recurrent droughts, and climate change is expected to make dry spells longer and more frequent. Yet California has built only one large desal plant (Carlsbad) and has rejected others, mainly over environmental concerns and cost. With Colorado River supplies plummeting and Sierra Nevada snowpack no longer reliable, the state is under pressure to augment its water portfolio. Passive desalination could be a game-changer here: coastal cities could deploy fields of floating desal units off their shores or near existing coastal facilities (like power plant outfalls, which already disturbed some marine area). Regulators who balked at RO might look more kindly on a zero-emission, low-impact alternative. The permit pathway would likely involve the California Coastal Commission – which has jurisdiction over coastal developments – but if environmental studies show minimal impact, approval is more likely. One can imagine pilot projects off the coast of Southern California, where demand is high and local water resources are low. Even supplying a fraction of municipal demand with passive desal could prove invaluable during drought emergencies. Moreover, California is exploring innovative water projects under Governor Newsom’s water resilience portfolio; a partnership to test Manhat’s technology in, say, San Diego or Santa Barbara could fit into those plans. There’s also the possibility of using passive desal to help remediate hyper-saline inland water bodies like the Salton Sea (in California’s Imperial Valley): placing units on the Salton Sea could extract fresh water (for nearby farms or dust control) while slowly reducing the Sea’s volume and salinity without large evaporation ponds or expensive treatment – effectively a controlled shrinkage with useful water as a byproduct. This kind of application blurs the line between desalination and environmental management, showing the tech’s flexibility.
- Arizona: Landlocked Arizona doesn’t have the ocean, but it exemplifies the Southwest’s water woes. Earlier in 2023, Arizona announced it would halt new housing construction in parts of the Phoenix area due to insufficient groundwater – a stunning development in a booming region. Projections show a 4.86 million acre-foot water shortfall in Phoenix over the next century. To close this gap, Arizona is considering everything from recycled wastewater to importing desalinated water from elsewhere. In fact, Arizona has contemplated partnerships with Mexico to build a desal plant on the Sea of Cortez (Gulf of California) and pipe the water north – a complex, costly proposition. Manhat’s floating desal could offer a different angle: smaller-scale desal in the Gulf of California or Salton Sea, or even deployment on large lakes and reservoirs. Arizona has abundant sunshine, so a solar-driven technology fits its ethos (the state is a leader in solar power). While Arizona itself might not deploy units (since it lacks coastal jurisdiction), Arizona-based investors or water agencies could pilot Manhat units through binational agreements in Mexico. The federal government might facilitate this under cross-border water treaties or through funding of pilot projects in partnership with Mexico’s water authorities – a sort of desal diplomacy. Additionally, Arizona has many brackish groundwater sites; conceivably, large evaporation ponds currently used for irrigation drainage could be retrofitted with floating stills to reclaim some water. The state’s focus is more on brackish desal (El Paso, on the AZ-TX border, runs the world’s largest inland desal plant for brackish water), but passive tech could potentially be applied to high-salinity brackish ponds, with the same advantage of not needing power.
- Texas: Texas is ”going big on desalination”, as one headline put it. The state has over 50 small desal plants (mostly treating brackish water) and is actively planning for large seawater desal facilities on the Gulf Coast. Corpus Christi, for example, has a 30 MGD Gulf desal plant in the works slated for 2028. Texas sees desal as key to supporting its growing cities and industrial sector (petrochemical plants, LNG terminals, etc.) especially as drought and overuse deplete rivers and aquifers. Unlike California, Texas’s regulatory environment is more permissive – the state even granted a permit for a desal plant intake that the EPA is now questioning on environmental grounds. This suggests Texas is eager to push desal projects through, even if federal regulators tap the brakes. Manhat’s technology could find a welcome mat in Texas for both economic and environmental reasons. Texas has a vast coast and many sunny, shallow bays that could host floating desal units. Coastal communities that currently rely on limited groundwater or long pipelines might opt for a local passive desal source to boost resilience against drought or hurricanes (which can disrupt pipelines and power – passive units would just sit through a power outage unaffected). There’s also synergy with Texas’s interest in offshore innovation: the state has been exploring offshore wind in the Gulf, and its ports service oil rigs – they have the marine engineering expertise to deploy and maintain offshore equipment. Dual-use possibilities are intriguing: for instance, a Gulf aquaculture farm (Texas is considering offshore fish farming as well) could integrate freshwater stills to provide clean water for hatchery operations or to periodically flush fish enclosures (some aquaculture operations use brief freshwater baths to treat marine fish for parasites). Texas also has coastal wildlife refuges suffering from hyper-saline conditions due to reduced inflows; placing desal floats in those areas could create fresher refuges for certain species without building energy-intensive facilities in ecologically sensitive zones. The state water plan even provides funding for innovative water supply projects – if pitched correctly, a pilot array of Manhat devices in Texas waters could secure support, showing how to get drought-proof water with no grid burden (a plus for Texas’s often-strained electric grid).
- Florida: The Sunshine State paradoxically gets plenty of rain, yet some regions face dry-season water shortages and saltwater intrusion into aquifers from over-pumping. Florida built the Tampa Bay Desalination Plant (25 MGD) to diversify its water supply, and despite early hiccups, it’s now an important drought-proof source for the region. Florida’s experience shows both the promise and pain of desal: it provides about 10% of Tampa’s water reliably, but maintaining it is costly and it was over budget and delayed. A passive approach might avoid some of those issues. Florida’s warm climate and mostly calm seas (aside from hurricanes) are conducive to solar desal. The state also has many coastal islands and communities not on the main grid – for example, the Florida Keys, which currently rely on a pipeline from the mainland for fresh water. A series of floating stills around the Keys or other islands could offer a decentralized backup supply, increasing resilience if the pipeline fails or during extreme drought when mainland supplies are low. Moreover, Florida has extensive coastal aquaculture in the form of shellfish (oyster and clam farms). While shellfish don’t need fresh water, the concept of dual-use platforms could apply: oyster farmers could attach a few desal units to their leases, producing a trickle of fresh water that might be used for cleaning equipment or even offering potable water for farm workers on their offshore platforms. Florida’s environmental agencies might appreciate that passive desal would not worsen the already sensitive estuarine environments (like Tampa Bay or Indian River Lagoon) where salinity balance is crucial. If anything, carefully used, it might help by providing a bit of fresh water that could be directed to marshes or used to mitigate salty discharges. For instance, during drought, the Everglades and associated wetlands suffer from too little freshwater flow – it’s fanciful, but one could imagine a large field of solar stills in a brackish lake feeding a trickle of fresh water into a critical marsh to keep it hydrated. Regulation in Florida for on-water structures would involve state environmental departments and water management districts, but Florida has shown a willingness to innovate (they’re leaders in potable water reuse and stormwater harvesting). An appealing aspect might be that passive desal aligns with Florida’s push for renewables and sustainability – it could be marketed as “freshwater from sunshine” in the Sunshine State.
In all these states, a key driver is the drought trends and unmet demand. The Western US is grappling with dwindling snowpack and over-allocated rivers. In the East (Florida), the issue is more about growth and seasonal imbalances. Water pricing is creeping upward as scarcity increases; in California’s Central Valley, farmers have paid hundreds of dollars per acre-foot for water transfers, and urban agencies have considered expensive emergency options (like Los Angeles eyeing ocean desal again after decades). Where water prices are high and expected to climb, a solution like passive desal becomes more economically viable. Importantly, unmet industrial demand is also a factor. Industries such as semiconductor manufacturing, data centers, and power plants require reliable water even during droughts. For example, Arizona’s burgeoning semiconductor factories (a critical, water-intensive industry) could invest in off-site desal (in Mexico or elsewhere) to secure a portion of their water needs independently of municipal sources. Similarly, petrochemical industries on the Gulf Coast could deploy passive desal floats to supply process water or cooling water, reducing their draw on local freshwater and easing community tensions over water use. The ability to deploy offshore, away from crowded freshwater systems, creates a new avenue to supply these industries. Climate-focused investors might find this particularly attractive as they seek to invest in solutions that both mitigate climate impact and adapt to it – passive desal does both by providing new water (adaptation) with no emissions (mitigation).
Regulatory Hurdles and Pathways
While Manhat’s technology dodges many environmental pitfalls, it will still face a regulatory gauntlet – albeit a different one than land-based plants. Policymakers should anticipate what changes or guidance might be needed to smooth the way for these novel systems:
- Offshore Jurisdiction: In U.S. waters, anything beyond 3 nautical miles offshore (for most states) falls under federal jurisdiction, primarily the Bureau of Ocean Energy Management (BOEM) for leasing space on the Outer Continental Shelf. BOEM has established processes for offshore oil, gas, and wind energy leases, but offshore desalination is not yet a defined category. A large installation of desal floats might require a BOEM lease or at least a right-of-use agreement. Policymakers could direct BOEM to create a streamlined category for “marine hydrokinetic and desalination devices”, similar to how wave-energy prototypes are handled, to ensure these projects aren’t stuck in bureaucratic limbo. If projects are within state waters (inside 3 miles), then state agencies and possibly state land commissions would have a say. For instance, the California State Lands Commission handles leases of submerged lands – they would need to permit anchors or mooring areas for a desal array. Clarity in jurisdiction – whether these are treated as aquaculture equipment, marine energy installations, or something new – will be needed. One possible approach is to treat floating desal units akin to navigational buoys or research buoys, which have relatively light permitting if they don’t unreasonably interfere with navigation or fishing.
- NOAA and Environmental Review: The National Oceanic and Atmospheric Administration (NOAA), through its National Marine Fisheries Service, will be interested in any potential impacts on marine life. While passive desal units have no toxic discharges, NOAA might study whether large arrays alter habitat or harm any protected species. For example, could sea turtles be confused by or entangled in the structures? Could a desal array in the Gulf of Mexico affect a nearby coral reef by changing local salinity or light? These are likely minor issues, but under laws like the Endangered Species Act and Marine Mammal Protection Act, any federal action (like BOEM issuing a permit) requires consultation on wildlife impacts. Fortunately, the mitigation for passive units should be straightforward – e.g., ensuring the units are spaced to allow fish passage, using materials that don’t leach, avoiding critical habitat areas – nothing as intractable as dealing with impingement or brine toxicity. NOAA also has a role in coastal zone management; state coastal agencies (like California’s Coastal Commission, or Florida’s Department of Environmental Protection) work with NOAA to enforce Coastal Zone Management Act provisions. These bodies may need to update their coastal management plans to explicitly encourage sustainable offshore water production projects, so that such projects are viewed favorably during consistency reviews.
- Coastal Commissions and Local Permitting: In places like California, even an offshore project can trigger review by the Coastal Commission if it affects the coastal zone (views, onshore facilities, etc.). Engaging early with such commissions to highlight the minimal footprint and high necessity of the project will be key. The very factors that sunk Poseidon’s project – marine impact and cost – would largely be neutralized with Manhat’s approach, giving commissions political cover to approve. However, agencies will still consider visual impact, public access, and cumulative effects. It may be wise to start with a pilot demonstration: for instance, deploy a dozen units as a pilot and monitor their output and environmental impact for a year or two. This low-risk trial can generate the data needed to satisfy regulators about scale-up. Public agencies (like water districts or state innovation funds) could partner in such pilots, sharing data transparently.
- Navigational Safety (Coast Guard): The U.S. Coast Guard will likely require that any clusters of floating desal units be marked on nautical charts and properly lit or signaled to prevent vessel collisions. This is standard for any offshore installation, but if we’re talking about potentially hundreds or thousands of small floating devices, it raises a practical question: how to make them visible to boats? Perhaps units on the perimeter of a field would have lights or radar reflectors, effectively creating a fenced zone, while interior units might not need individual lights. Coast Guard regulations on private aids to navigation would need to be considered. For policymakers, the task will be to coordinate maritime rules with the need to treat these as benign infrastructure. If offshore aquaculture pens and wind farms could be permitted (both of which involve many anchored structures), there’s precedent to follow.
- Water Quality and Rights: Interestingly, desalinated water produced offshore and brought to land might bypass some traditional water rights frameworks, which are usually about rivers and groundwater. However, states might need to define ownership of water produced from the sea. Likely, if a utility or company produces it, it’s theirs to use or sell (once onshore). One regulatory hurdle might be water quality standards – for potable use, the product water must meet EPA drinking water standards. Distilled water from the units will be pure, but the storage and handling must avoid contamination. Any pipeline bringing it ashore might be considered a public water system and fall under regulatory oversight for safety. These are routine concerns – engineers know how to keep water clean – but it means coordinating with state drinking water regulators.
In general, the regulatory environment can be navigated with planning, but policy support could greatly accelerate it. For example, federal funding for pilot projects (perhaps through the Department of Energy’s water desalination programs or the Bureau of Reclamation’s WaterSMART grants) could include directives to agencies to expedite permitting as a demonstration. The U.S. Department of Energy (DOE) is already funding offbeat desalination research – notably, it allocated $2 million in late 2024 for projects to harness wave energy for desalination, aiming to aid coastal communities and disaster relief with wave-powered RO units. This shows the federal interest in off-grid desal tech. Manhat’s solar stills fall into a similar innovative category, and could attract DOE or National Science Foundation grants. Policymakers might also consider legislative nudges: for instance, a bill to classify renewable-powered desalination as a “beneficial use” of ocean space and water nationally, giving it a clearer legal status.
Aquaculture and Dual-Use Opportunities
The prompt specifically highlights coastal aquaculture co-benefits, pointing toward a vision of dual-use systems that produce both water and food. Manhat’s own focus on floating farms (agriculture) is one example of dual-use: the same platform both grows crops and produces water for those crops. But we can extend this concept to aquaculture (fish, shellfish, seaweed):
Imagine a marine aquaculture park – an area offshore where fish are farmed in cages or shellfish on lines. These farms often need infrastructure like feeding barges, staff outposts, or storage. If we equip the area with desalination units, the fresh water produced could serve several purposes:
- Fish Husbandry: Many marine fish species can benefit from occasional freshwater baths to remove parasites or treat disease (for instance, salmon farms use wellboats with freshwater for sea lice treatment). Having a source of fresh water on-site, via solar desal units, would save transporting water from shore. It could also provide drinking water for personnel stationed offshore, or for cleaning equipment (nets, tanks) without corroding them with saltwater.
- Integrated Multi-Trophic Aquaculture (IMTA): This is a concept where multiple species are farmed together to utilize waste from one as input for another (e.g., fish waste fertilizes seaweed or shellfish). Fresh water isn’t typically part of IMTA, but one could envision a holistic system where desal units provide a small stream of fresh water that could, say, flush through a holding pond for juvenile fish or mix with feed. Also, if one were cultivating certain types of algae that prefer lower salinity, a mix of desalinated water could create optimal brackish conditions in a section of the farm.
- Salt and Mineral Byproducts: Although Manhat’s system doesn’t produce a separate brine stream, locally the water under each unit gets a bit saltier before diffusing. If there were a way to capture or utilize that (for example, by having the unit integrated with a shallow pond that can periodically be isolated), one could harvest sea salt or minerals as a co-product. Aquaculture operations could potentially use that salt for processing food products (many seafood products are packed in salt or brine). This is speculative, but it highlights that these systems blur the line between water production and resource production.
- Spatial Synergies: Aquaculture farms often have exclusion zones for boating around them for safety; desal units could sit within those zones without causing additional exclusion, thus sharing ocean space efficiently. They could even act as a sort of breakwater for fish pens, calming waves (if enough of them in an array) and improving conditions for the fish. Conversely, the presence of fish cages might actually help the desal units by deterring large marine animals or heavy debris that might damage them.
- Food-Energy-Water Nexus: By combining aquaculture with renewable-powered desalination, one could market the output as especially sustainable – fish grown with minimal environmental impact and water produced with zero carbon. This might attract premium pricing (much as “sustainably farmed” seafood does). For policymakers, encouraging such nexus projects could yield multiple benefits (food security, water security, economic development) from one initiative. NOAA, which is promoting offshore aquaculture, and DOE, which promotes marine energy, might find common cause in an integrated pilot project.
It’s worth noting that some countries are already exploring off-grid desal for aquaculture. In Chile and Norway, salmon farms in remote fjords have tested solar-powered RO units to get fresh water for their operations. Manhat’s approach could be even simpler to deploy in those contexts (no fuel, just floats). As the aquaculture industry grows – potentially into a multibillion-dollar offshore enterprise – having freshwater on-site could be a competitive advantage.
The Scale of the Opportunity
Stepping back, how big could this be? The global water market was valued at approximately USD 351.9 billion in 2024 and is projected to reach USD 674.8 billion by 2033, growing at a 7.5% compound annual growth rate (CAGR), encompassing supply infrastructure, treatment, and services. Desalination is a growing slice of that: the global desalination market is valued around $20–30 billion today and expected to double within a decade, driven by water scarcity and urban growth. Traditional desalination companies (and their investors) have long looked at rich, arid regions – the Middle East, parts of the U.S., Australia, etc. – as key markets. But those conventional methods come with baggage, as we’ve outlined. A technology that can provide desalinated water with lower capital requirements and minimal environmental hurdles could unlock demand in places that until now found desalination impractical or undesirable.
Consider small island developing states or remote coastal communities globally: they often rely on diesel-powered generators for electricity and diesel-fueled desal units or imported bottled water. Manhat’s units could offer a lifeline of fresh water without fuel dependency, and at a community scale that avoids large infrastructure. For investors focusing on climate adaptation, funding deployments in such areas could not only be profitable (if structured as a water-as-a-service model) but also have huge social impact, aligning with ESG criteria.
Within the U.S., the opportunity may first materialize in public-private partnerships. Water agencies in California or Texas could partner with technology providers to pilot these systems, perhaps using federal grants. If successful, it could spur a procurement program – much like some utilities have programs to subsidize rooftop solar, we could see programs to deploy floating desal units in strategic locations. Private capital, meanwhile, might see a chance to own and operate desal fleets that sell water to governments or industries. Climate tech investment has poured into energy storage, carbon capture, etc., but water – especially desal – is now getting attention as water scarcity joins the list of climate emergencies. Manhat itself is a startup that has won innovation awards and grants (about $200,000 in grants so far), but scaling to a global solution will require much larger capital infusions for manufacturing and deployment. Investors with a long view might compare the trajectory to that of solar PV: initially more expensive than the incumbent technology (fossil power), but with a clear path to cost reduction and massive scale. Similarly, passive desal may start as a niche or supplemental source, but given the trend lines – persistent droughts, rising water prices, public pushback on big desal plants, and the ever-increasing value of carbon-free solutions – it could become a mainstream tool in water strategy.
Conclusion: A Paradigm Shift in the Making
Manhat’s floating desalination technology represents a potential paradigm shift in how we think about sourcing water. Instead of a mega-project, it offers a modular, eco-friendly approach – essentially tapping the ocean-atmosphere interaction that the planet already uses to create rain, and doing it on demand where needed. For policymakers, this could be a way to break the stalemate that often occurs between urgent water needs and environmental protection. The technology bypasses the choke points (high energy use, marine habitat damage, huge upfront costs) that have made seawater desalination a solution of last resort in the US. And for climate investors, it aligns with the dual goals of mitigation and adaptation: providing critical infrastructure for a drier future without contributing to the problem.
Of course, due diligence is required. It’s important not to view Manhat’s system as a magical fix that can simply replace all existing water projects. Practical pilots should be launched in U.S. waters to gather data on production rates, maintenance needs, and integration with local water systems. Economic modeling is needed to firm up the cost per acre-foot under various scenarios. We also have to consider public perception – early successes will build the narrative that this is a viable and desirable solution, whereas any high-profile failures could set it back. In that sense, careful implementation is as important as the technology itself.
Policymakers can help by creating an enabling environment: fast-track permits for experimental deployments, grants or innovation prizes to encourage refinements (perhaps improved materials, or smart sensors to monitor each unit’s output in real time), and frameworks for utilities to purchase water from unconventional sources. Inter-agency cooperation (DOE, DOI/BOEM, NOAA, EPA, and state agencies) will be needed to address the cross-cutting nature of offshore water production.
What’s at stake is significant. Water scarcity is no longer a distant threat; it’s here. The American West’s megadrought and decisions like Arizona’s building moratorium show that old approaches (groundwater mining, ever-longer pipelines) are reaching their limits. As these stresses grow, the opportunity cost of inaction is huge – measured in fallowed farmland, restricted growth, ecological collapse, and even potential conflicts over water. Conversely, the upside of a solution like passive desalination is transformative: it opens up a new, virtually untapped source (the ocean) in a manner that is sustainable. It could allow coastal agriculture to thrive without drawing down rivers, enable cities to grow without siphoning their hinterlands, and provide a buffer in dry times that doesn’t exacerbate climate change.
In an editorial in one of the major newspapers, one might conclude by invoking an analogy: just as renewable energy micro-grids are changing the energy sector, renewable water micro-grids might change the water sector. Manhat’s floating stills, scattered across a bay or tethered along a coast, could become the water equivalent of solar panels – a visible testament to innovation and resilience. The Best Practices Award at Expo 2025 is a nice trophy, but the true prize will be if this technology can help secure water for millions of people in an affordable and sustainable way. Policymakers and investors have a chance to take this UAE-born innovation and nurture it on U.S. shores, demonstrating global leadership in tackling water scarcity. It’s a bet on a future where solving our water challenges doesn’t come at the expense of our planet, but rather works in harmony with it – a future that, in light of climate change, we desperately need to build.