A New Star in the Fusion Firmament
In late March 2025, a small fusion startup based in Knoxville, Tennessee made a big announcement that reverberated through the energy world. Type One Energy unveiled “Infinity Two,” the design basis for its first fusion power plant – and with it, the most comprehensive scientific validation ever published for a commercial stellarator reactor. In plain terms, this under-the-radar company claims it has cracked a key piece of the fusion puzzle: a realistic, unified design for a working fusion pilot plant, vetted by peer-reviewed studies and ready to move from theory into engineering. This isn’t a flashy lab experiment or a sci-fi sketch – it’s a detailed blueprint that a major U.S. electric utility is already eyeing as a potential power source by the mid-2030s.
Fusion energy has long been the “holy grail” of clean power – promising virtually limitless, carbon-free electricity by harnessing the same process that makes stars shine. Yet it’s also been notorious for always seeming at least 20 years away. What makes Type One Energy’s news different? For one, Infinity Two isn’t just a concept on paper; it’s backed by seven rigorous scientific papers published in the Journal of Plasma Physics, laying out the physics and engineering parameters in unprecedented detail. And rather than the more common tokamak fusion reactor design pursued by many competitors, Type One is betting on the stellarator – a twisty, pretzel-shaped fusion device that for decades was an underdog in fusion research. In doing so, the company is marrying cutting-edge computational physics with an unconventional reactor design that could offer big advantages in reliability and continuous operation.
Equally significant, Type One has secured a partnership with the Tennessee Valley Authority (TVA), the large federal electric utility in the U.S. Southeast, to explore building its fusion plant in the region. This makes Type One the first private fusion firm to team up with a utility on a potential plant site – a sign that its design has progressed beyond laboratory daydreams. “Why are we the first private fusion company with an agreement to develop a power plant for an energy utility? Because we have a design anchored in reality,” says Christofer Mowry, CEO of Type One Energy. Mowry’s confidence stems from grounding Infinity Two’s design in hard-earned lessons of what a power station actually requires: robust operations, maintainability, and economics, not just scientific novelty. In his words, “this isn’t about designing a science project”. It’s about proving fusion can finally become a workhorse on the electric grid.
From Donuts to Pretzels: Stellarators vs. Tokamaks
Most of the world’s fusion efforts – from the giant $25 billion ITER project in France to flashy startups in the U.S. – rely on the tokamak, a ring-shaped device that uses powerful magnets and an electrical current in the plasma fuel to confine and heat it. Tokamaks have achieved the best fusion performance so far and “have come closest to achieving fusion conditions” as one veteran physicist notes. But tokamaks also have well-known drawbacks: they typically operate in pulses (not continuously) and suffer from disruptions, sudden losses of control of the super-hot plasma. Those disruptions can damage the device and make steady power generation a challenge. As Dr. David Gates, a plasma physicist at Princeton Plasma Physics Lab (PPPL), bluntly put it, tokamaks face instabilities that are hard to avoid when pushing to reactor conditions. “Generally, tokamaks operate in a pulsed mode and are subject to disruptions. A practical fusion reactor will require continuous operation… ‘Stellarators offer the best possibility for steady-state operation,’” Gates says.
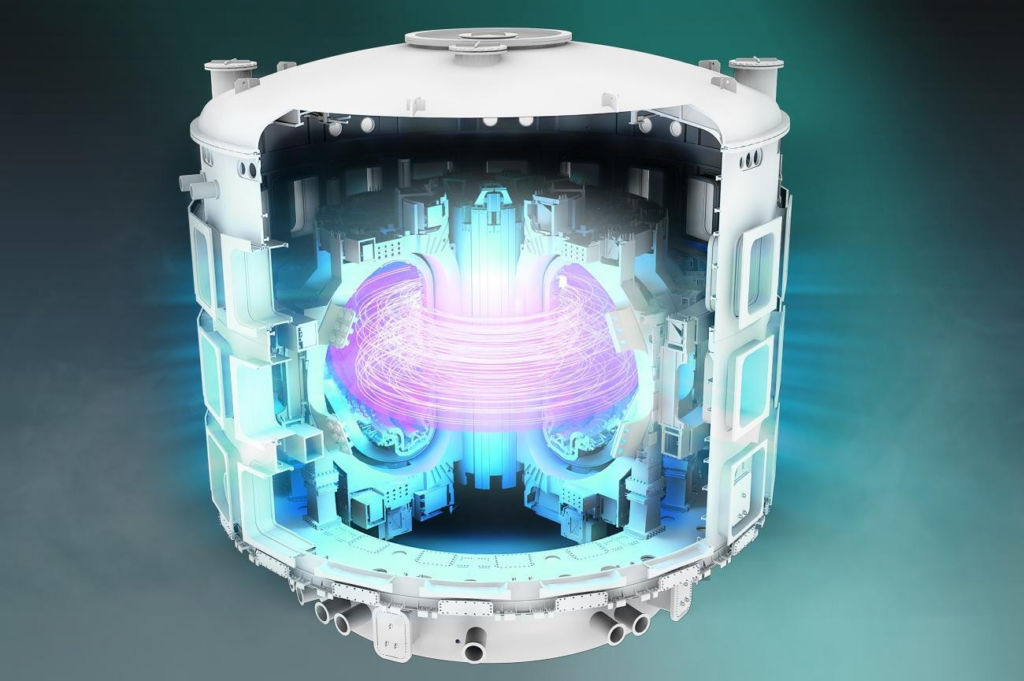
Stellarators, by contrast, take a very different engineering approach. Instead of driving a strong electric current through the plasma to shape it (as tokamaks do), a stellarator uses only external magnets – intricately twisted coils surrounding the vacuum chamber – to confine the plasma in a carefully contorted magnetic cage. The result is a device that looks like a toroidal Möbius strip or a helix looping around on itself. This complexity had a purpose: by “twisting” the magnetic field lines in a pretzel-like configuration, a stellarator can keep the hot plasma fuel stable without needing the pulsing transformer at a tokamak’s core. In practical terms, that means a stellarator can, in theory, run continuously for long periods – a huge plus for a power plant. It also means no risk of sudden disruptive instabilities caused by internal currents, since the stellarator’s plasma isn’t leaning on an internal current to stay confined. The trade-off historically was that stellarators were fiendishly hard to design and build. Their three-dimensional magnetic geometry defied early analytical formulas, and their twisted coils were a nightmare for mid-20th-century manufacturing. Consequently, stellarators “took a back seat” to the simpler donut-shaped tokamaks for decades.
That picture has started to change, leading some to speak of a “stellarator renaissance.” Over the last 10–15 years, advances in supercomputing and optimization algorithms have allowed scientists to precisely calculate coil shapes and magnetic fields that confine plasma almost as well as a tokamak’s symmetric field. In 2015, Germany fired up the world’s largest stellarator, the Wendelstein 7-X, to test these ideas. By early 2023, Wendelstein 7-X had demonstrated confinement times and plasma performance on par with top tokamaks, all in steady-state operation, and even achieved up to 30 minutes of continuous plasma discharge in test runs. Perhaps most importantly, it proved that modular superconducting magnet coils – shaped in bizarre curves – could be built and operated reliably at a large scale. “Recent developments, including the impressive performance of W7-X in Germany and results from [Japan’s] LHD stellarator, have created a renaissance of interest in these twisty machines,” PPPL noted in 2022. Researchers also found clever ways to simplify future stellarators, such as using small permanent magnets to fine-tune the field instead of overly complex coils.
Type One Energy is very much riding this wave. In fact, the company’s scientific pedigree is rooted in stellarator research – its chief scientist is Dr. Chris Hegna, a University of Wisconsin physicist renowned for stellarator theory. The Infinity Two design explicitly leverages the “inherently favorable operating characteristics” of highly optimized stellarator technology demonstrated by W7-X. In other words, they took the lessons of W7-X’s success – stable plasmas, no disruptions, long operation – and pushed them further with modern computing. Over two years, Hegna’s team ran intensive simulations on U.S. Department of Energy supercomputers (including Oak Ridge’s cutting-edge “Frontier” exascale machine) to iteratively refine the reactor shape. The outcome was a design that balances the many competing requirements of a real power plant: plasma performance, startup and fueling, heat exhaust, tritium fuel self-sufficiency, maintainability, and even economics. Achieving all these at once is a tall order – but Type One claims to have found a sweet spot. Per Helander, head of stellarator theory at Germany’s Max Planck Institute (and a leading mind behind W7-X), reviewed the Type One studies and remarked that they “set new standards for the fidelity and confidence level” in stellarator reactor design.
What does Infinity Two look like on paper? It’s a deuterium-tritium fueled stellarator that produces 800 MW of fusion power, delivering around 350 MW of electric output to the grid. (For scale, that’s enough to power roughly 300,000 homes, akin to a small fission nuclear plant or a large gas-fired plant.) The design features a resilient plasma configuration that remains stable over a broad range of operating conditions. Advanced “island divertors” are incorporated to safely exhaust helium ash (the spent fusion fuel), and there’s ample space for a surrounding blanket that both shields the reactor structure and breeds tritium to sustain the fuel cycle. By building in tritium breeding capability, the plant could generate its own supply of this rare fuel from lithium, an essential step for practical D-T fusion. The company emphasizes that these features come with “conservative design margins” and very low levels of turbulent heat loss in the plasma – meaning they aren’t banking on exotic, unproven physics. All of this gives Type One “high confidence that this essential physics solution provides a good baseline” for their pilot plant.
Equally important for a power producer, Infinity Two is being engineered for maintainability and economic viability. Stellarators historically were not only tricky to design, but also to service – their complex geometry can complicate replacing components. Type One says it has “architected a maintenance solution” to support good capacity factor (uptime) and a competitive cost of electricity. The design also considers regulatory and construction requirements to keep the overnight capital cost reasonable. In essence, the company is trying to anticipate the real-world headaches of building and operating a fusion plant, not just the plasma physics. It’s a strikingly pragmatic mentality in a field that, until recently, was more about chasing scientific milestones than worrying about, say, how to change out a radioactive blanket module every few years.
Building a Bridge from Coal to Fusion in Tennessee
One of the most concrete signs that Type One Energy’s approach is being taken seriously is its partnership with the Tennessee Valley Authority. In February 2024, Type One, TVA, and Oak Ridge National Laboratory announced Project Infinity, a collaboration to advance fusion energy in East Tennessee. As part of this project, Type One will actually build a prototype stellarator called “Infinity One” at TVA’s retired Bull Run Fossil Plant site in Clinton, TN. Bull Run, once a coal-burning power plant, is slated to become home to a next-generation fusion experiment – a powerful symbolic shift from old energy to new. The plan is to begin construction of Infinity One as soon as 2025 (pending environmental reviews and permits). This device won’t be a full power plant, but a high-field stellarator prototype to validate key technologies and design features for the later Infinity Two reactor. In particular, Infinity One will test aspects of operating efficiency, reliability, and maintenance procedures in a smaller package, de-risking the eventual scale-up.
By moving its R&D operations to the TVA site, Type One is also tapping into a local talent pool and infrastructure. The company is relocating its headquarters from Madison, Wisconsin to East Tennessee, aiming to create over 300 high-skilled jobs in the next five years. The state of Tennessee jumped in as well – Governor Bill Lee’s administration awarded initial funding from a state “Nuclear Energy Fund” to kickstart Project Infinity. For TVA, which supplies power to seven states, exploring fusion aligns with its mission to provide affordable, reliable electricity. The utility sees the 350 MWe Infinity Two plant as a possible “complementary source of baseload generation” in its mix by the mid-2030s. Notably, TVA is interested in whether a fusion plant could reuse retiring fossil plant sites – taking advantage of existing grid connections and land where coal plants once stood. If Infinity Two works as hoped, TVA could replace a shuttered coal unit with a fusion unit, instantly cutting emissions while using the same transmission lines and support facilities. It’s an elegant vision: turning yesterday’s polluting infrastructure into tomorrow’s clean power hub.
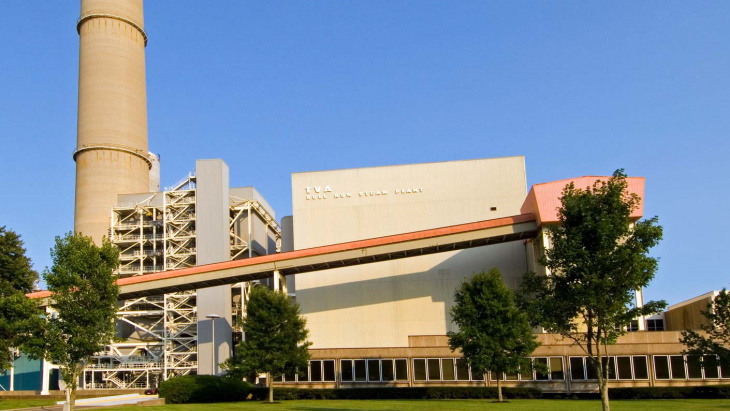
Under a cooperative agreement signed in early 2025, TVA and Type One are conducting siting studies, environmental assessments, and licensing preparations for Infinity Two, while also hammering out project plans and financing options. This agreement expands the earlier ORNL partnership and is notable for how hands-on the utility is getting. TVA’s expertise in building and running large power projects (including nuclear plants) will inform the fusion plant’s development. In parallel, TVA has opened up its Power Service Shop facilities in Muscle Shoals, Alabama to Type One, helping the startup develop its supply chain and manufacturing techniques for stellarator components. In short, a federal utility, a national lab, a state government, and a private fusion company are aligning forces – a coalition that underscores both the excitement and the seriousness around this effort.
The mid-2030s timeline for Infinity Two also dovetails with U.S. national goals. The Biden Administration in 2022 set forth a “bold decadal vision” to accelerate fusion energy development, calling for the construction of a pilot fusion plant within 10 years (by the early 2030s) as a milestone toward commercial fusion. Type One’s plan puts TVA’s region on track to potentially host one of the first functioning fusion pilot plants in the country, right on that schedule. If successful, it could make the Tennessee Valley a flagship example of fusion-powered clean energy – and give the U.S. a leadership position in a field that is becoming increasingly global and competitive.
The New Fusion Landscape: Stellarators and Beyond
Type One Energy is moving in a space that’s suddenly bustling with fusion startups, each taking a distinct path to try to achieve the long-sought breakthrough of net energy gain and practical power generation. For decades, fusion research was dominated by government-funded projects like tokamaks in national labs and the international ITER collaboration. But in the last 5–10 years, private companies – often venture-backed – have raised billions of dollars to develop smaller, faster, and sometimes radically different fusion systems. This entrepreneurial surge has injected both fresh optimism and a healthy dose of competition into the fusion race. How does Type One’s stellarator approach stack up against the leading competitors? Let’s compare their strategies, timelines, and recent milestones:
Helion Energy: Fusion in a Flash – On the opposite coast from Type One, in Everett, Washington, Helion Energy has been making waves with an approach that is as unconventional as its ambitious timeline. Helion isn’t using a tokamak or a stellarator at all – it’s pursuing a pulsed, linear method known as a field-reversed configuration (FRC). Essentially, Helion’s device shoots two rings of magnetically contained plasma from opposite ends of a long tube, slamming them together at incredible speeds in a central chamber. The collision compresses and heats the plasma to fusion conditions – akin to an “internal combustion engine” for plasma, where each pulse is like a tiny detonation driving a piston. When fusion occurs, Helion does something very clever: the fusion reaction’s energy causes a rapid expansion of the plasma’s magnetic field, which pushes back on the coils that launched it, inducing an electric current. That surge of current is captured directly as electricity – no steam, no turbines. In principle, this direct energy recovery could make the system highly efficient, since it sidesteps the usual heat-to-steam conversion losses of power plants. “Because the system harvests electricity from [the] magnets instead of generating steam to spin a turbine, it should be more efficient, lowering the bar to break even,” explains Helion CEO David Kirtley. If everything works perfectly, each fusion pulse would generate more electricity than the machine consumed to fire the plasma, achieving net gain and directly outputting power to the grid.
Helion’s approach is bold not only in design but also in timeline. The 12-year-old company has already built six prototypes, each improving on the last, and flipped the switch on its seventh prototype, dubbed “Polaris,” in late 2024. Polaris is expected to be the first fusion device to ever produce electricity (even if just in experimental bursts) – a milestone Helion hopes to claim imminently. The company has attracted intense interest partly due to its high-profile backer, Sam Altman (the tech investor known for OpenAI), who has personally invested at least $375 million and serves as executive chairman. In 2023, Helion grabbed headlines by signing the world’s first fusion power purchase agreement (PPA): a deal to sell electricity to Microsoft by 2028. Under that agreement, Helion aims to deliver 50 MW of power from its first commercial fusion unit to Microsoft’s cloud datacenters, as early as five years from now. This timeline – providing grid power well before any other fusion effort – is extremely aggressive, and many experts remain skeptical. But it has lit a fire under the industry. Helion’s recent funding reflects the urgency: in January 2025, it raised another $425 million (led by SoftBank’s Vision Fund), bringing its valuation to about $5.4 billion. The company now employs around 450 people and is scaling up manufacturing of custom components (like 50,000 high-power thyristor chips and 6,000 capacitors for each machine) to meet its goals. With its rapid-fire pulses – the next-gen design aims for perhaps 10 pulses per second or more – Helion essentially wants to turn fusion shots into a continuous buzz of electricity. It’s a strategy that trades the steady-state elegance of a stellarator for a brute-force, repetitive approach more akin to an engine or a high-frequency laser. If it can overcome the massive engineering challenges (maintaining components through billions of pulses, and keeping each plasma shot consistent and efficient), Helion could potentially be the first to put fusion power on the grid – even if only for fractions of a second at a time initially.
Helion’s prototype fusion device “Polaris” generates a flash of pink plasma during a test. The Washington-based startup uses a pulsed approach: two plasma rings collide in the center of the machine, and the fusion energy is captured directly as electricity via changing magnetic fields. Helion’s audacious goal is to have a 50 MW fusion unit supplying the grid by 2028, thanks in part to a landmark power supply deal with Microsoft. The company has raised over $900 million from investors including Sam Altman, and it’s already testing high-power components in pursuit of net energy gain.
Commonwealth Fusion Systems: High-Field Tokamak, High Hopes – In Massachusetts, another fusion contender with MIT roots is taking a very different path: sticking with the proven tokamak formula, but turbocharging it with new magnet technology. Commonwealth Fusion Systems (CFS) was founded in 2017 by a team from MIT’s Plasma Science and Fusion Center, and it has become a darling of investors looking for a more conventional, physics-backed bet on fusion. CFS’s approach centers on a compact tokamak called SPARC, which will use high-temperature superconducting (HTS) magnets to achieve ultra-strong magnetic fields. In 2021, CFS demonstrated a record-breaking magnet that reached 20 tesla – the strongest magnetic field of its kind. These new HTS magnets (made from novel superconductors like rare-earth barium copper oxide) allow a tokamak to confine plasma much more tightly, enabling a smaller device to achieve the same performance that previously required a machine the size of a building. With this magnet milestone, CFS attracted a staggering $1.8 billion Series B funding round in late 2021, led by investors such as Tiger Global and Bill Gates’s Breakthrough Energy Ventures. This war chest – one of the largest single raises in clean energy startup history – is funding the construction of SPARC and the design of an ensuing pilot plant called ARC.
CFS’s timeline is ambitious but a tad more measured than Helion’s. The company began building a new campus in Devens, MA, where SPARC’s tokamak is being assembled, and it aims to have SPARC achieve net energy from fusion by 2025-2026. “Net energy” here means the plasma in SPARC should produce more fusion power than the heating power put into it – a scientific break-even expected to be on the order of Q ≈ 2 (twice as much out as in). That would be a historic first for a magnetically confined plasma. CFS has published detailed physics studies indicating SPARC will indeed reach this goal. If SPARC succeeds, it will pave the way for the ARC plant in the early 2030s, which is planned as the world’s first commercial fusion power station. The ARC design is targeting around 400 MW of electricity output (similar to Infinity Two’s 350 MWe) and will leverage the lessons from SPARC to be a fully functional generator connected to the grid. In December 2024, Commonwealth Fusion announced it had selected a site in Chesterfield County, Virginia – just outside Richmond – to build the ARC plant, in partnership with the local utility Dominion Energy. Dominion is providing the site and grid access under a collaborative agreement (though not financing the project), and CFS will finance and build the plant itself. This move underscored how far the company has progressed: from an MIT classroom idea in 2012 to planning a grid-scale power plant deployment by ~2032. Dennis Whyte, the MIT professor who co-founded CFS, called it “a watershed moment for fusion… It sets the pace in the race toward commercial fusion power plants. The ambition is to build thousands of these and change the world”.
Technically, CFS’s tokamak strategy carries forward much of the mainstream fusion knowledge from projects like ITER, but turbocharged with private-sector agility and the magnet innovation. The SPARC tokamak will be compact (about 3 meters major radius) but with an extremely high magnetic field (around 12–13 tesla on the plasma, thanks to those HTS coils). That high field is the “secret sauce” allowing SPARC to achieve a burning plasma at smaller scale. The design is relatively conservative beyond the magnets – conventional deuterium-tritium fuel, a tokamak configuration similar to past machines, and short pulse operation (it won’t run steady-state, but long enough to reach equilibrium). The big question for CFS will be how to bridge from a pulsed net-energy experiment to a practical power plant. ARC is supposed to solve that: it will incorporate features like a breeding blanket for tritium, better heat exhaust systems, and (the team hopes) a method for handling the neutron loads and materials issues that come with an actual power reactor. Commonwealth Fusion’s leaders are optimistic that with SPARC’s results in hand by the late 2020s, they can finalize ARC’s engineering and have it delivering hundreds of megawatts to the grid by about 2035. They emphasize that fusion’s benefits – abundant fuel from seawater and lithium, no long-lived waste, zero carbon emissions – are within reach on that timeframe. “Fusion can significantly increase the pace at which the world cuts its carbon emissions… a commercial fusion plant is soon going to be within reach,” said Bob Mumgaard, CFS’s CEO. With over $2 billion raised to date and heavyweight backers like Bill Gates, Google, and major oil companies (Eni and Equinor invested early), Commonwealth Fusion Systems is often cited alongside Helion as a frontrunner in the fusion startup space – albeit with a very different style. If Helion is racing to show fusion sparks in a hurry, CFS is methodically building a sturdy torch, drawing on decades of tokamak experience and the might of superconducting tech.
TAE Technologies: Fusion Beyond D-T – Another veteran in the fusion startup world is TAE Technologies, based in California. TAE (short for Tri Alpha Energy, its original name) has been around since the late 1990s, making it one of the pioneers of private fusion R&D. Its approach is yet again distinct: TAE is working toward using advanced fuels like hydrogen-boron (p–¹¹B) that produce no neutrons – often called aneutronic fusion. The allure of p–B¹¹ fusion is that it generates only charged particles (helium nuclei), which could be converted to electricity directly and don’t activate reactor structures with neutron radiation. The catch? It requires extremely high temperatures, on the order of a billion degrees Celsius, far higher than D-T fusion. TAE’s strategy has been to pursue this long game in stages. Their current devices use a hydrogen isotope (deuterium) and helium-3 or proton fuels to progressively step up performance, employing a configuration called a field-reversed configuration (like Helion’s general plasma shape, an FRC) confined by a blend of magnetic fields and plasma particle beams. Over successive machines (each named after a scientist – e.g. “Norman” after Norman Rostoker, a company founder), TAE has set temperature and confinement records for FRCs. In 2022, the company announced that it had exceeded its performance goals on the Norman device by 250%, reaching plasma temperatures of over 75 million °C – an encouraging progress toward the hundreds of millions needed.
Armed with those results, TAE raised $250 million in mid-2022 from investors including Google and the oil giant Chevron, bringing its total funding to $1.2 billion to date. The infusion is financing TAE’s next machine, called “Copernicus,” which will be built in Irvine, California as the company’s sixth-generation reactor. Copernicus is intended to demonstrate net energy generation from D-T or D-He3 fusion, thereby validating the approach before the leap to hydrogen-boron fuel. The company’s CEO, Michl Binderbauer, has stated a goal “to begin commercialization of fusion by the end of this decade”. That suggests TAE hopes to have a prototype or pilot plant operating in the early 2030s, likely using D-T initially, with the ultimate aim of transitioning to aneutronic fuel cycles later. TAE’s long-term vision is a reactor named “Da Vinci” (following Copernicus) that would achieve p–B¹¹ fusion, producing clean power with only harmless helium as waste.
TAE’s approach is notable for its use of particle beams to stabilize the plasma. In the Norman reactor, for example, twin particle beams are injected into the FRC plasma to help maintain its stability and temperature. This “beam-driven” FRC concept is quite unlike the magnet-heavy tokamak or stellarator – it’s a hybrid of magnetic and inertial stabilization. The company also leverages machine learning heavily (through a long-standing collaboration with Google) to control and optimize its plasma shots in real-time. In terms of market positioning, TAE has been somewhat quieter in public timelines than Helion or CFS, perhaps reflecting its more research-intensive roadmap. But it has forged partnerships beyond energy: the firm spun off a subsidiary in medical diagnostics (using its accelerator technology for cancer treatment development) and another in electric power management – hedging its bets and generating interim revenue while fusion is still in development. TAE’s investors are a who’s-who as well, ranging from institutional capital (NEA, Venrock) to strategic partners. Notably, both Google and Chevron have put money in – Google for the AI and clean tech angle, and Chevron likely for a stake in a potential breakthrough that could revolutionize the energy landscape. “Fusion technology as a whole has the potential to be a scalable source of no-carbon energy and a key enabler of grid stability as renewables grow,” said Jim Gable, president of Chevron Technology Ventures, explaining the investment. TAE’s success would mean a fusion source that complements renewables without the neutron radiation issues of D-T fusion – a very attractive prospect, albeit further on the horizon. In the near term, all eyes will be on whether Copernicus (expected to come online mid-decade) can hit the benchmarks needed to prove out net energy gain.
Other Contenders – The fusion arena includes several other notable players and approaches. General Fusion, based in Canada (with backing from Jeff Bezos), is developing a unique magnetized target fusion system: it injects a magnetized plasma into a sphere of swirling liquid metal and then rapidly compresses the liquid with synchronized pistons to ignite fusion. General Fusion plans a large demonstration in the UK by the late 2020s, though it recently underwent leadership changes. Zap Energy in Washington state is pursuing a sheared-flow Z-pinch – an elegantly simple concept where an electric current compresses the plasma (pinch) and flow shear stabilizes it. Zap raised over $200 million and hopes to achieve fusion conditions in the next couple of years with a much smaller, electrode-based device. Inertial fusion startups are also emerging in the wake of the U.S. National Ignition Facility’s 2022 breakthrough success (where 192 lasers achieved a brief fusion ignition event). For example, Xcimer Energy and Focused Energy are new companies looking to turn laser fusion or pulsed laser-plasma fusion into a power plant concept, though they are at early stages. Even in the tokamak sphere, the U.K.’s Tokamak Energy (a private company) is building high-field spherical tokamaks with HTS magnets, similar in spirit to CFS. Tokamak Energy has raised over $200 million and was reportedly considering a SPAC merger to go public, though those plans have not yet materialized amidst shifting market conditions.
What’s remarkable is that dozens of companies worldwide – at least 44 firms as of 2023 – are now in the fusion race. They range from well-funded “unicorn” startups like CFS, Helion, and TAE to small university spin-offs. They’re pursuing an array of concepts, some leveraging breakthroughs in materials (like better magnets), others in computing (AI control, advanced simulations), and others in engineering (novel laser systems or inventive plasma configurations). This burgeoning ecosystem has attracted more than $6.2 billion in private investment cumulatively by mid-2023, up from $4.8 billion a year before. In the past year alone (2022–2023), over $1.4 billion of new funding flowed into fusion ventures, despite a generally tight venture capital environment. Four fusion startups – likely the leaders we’ve discussed – told researchers they believe they will have fusion power on the grid by 2030, and 19 companies predict doing so by 2035. That optimism may be inflated, but it signals a sea change in attitude: where fusion was once entirely a government-driven, very-long-term prospect, it’s now a dynamic commercial innovation space with real timelines and customers.
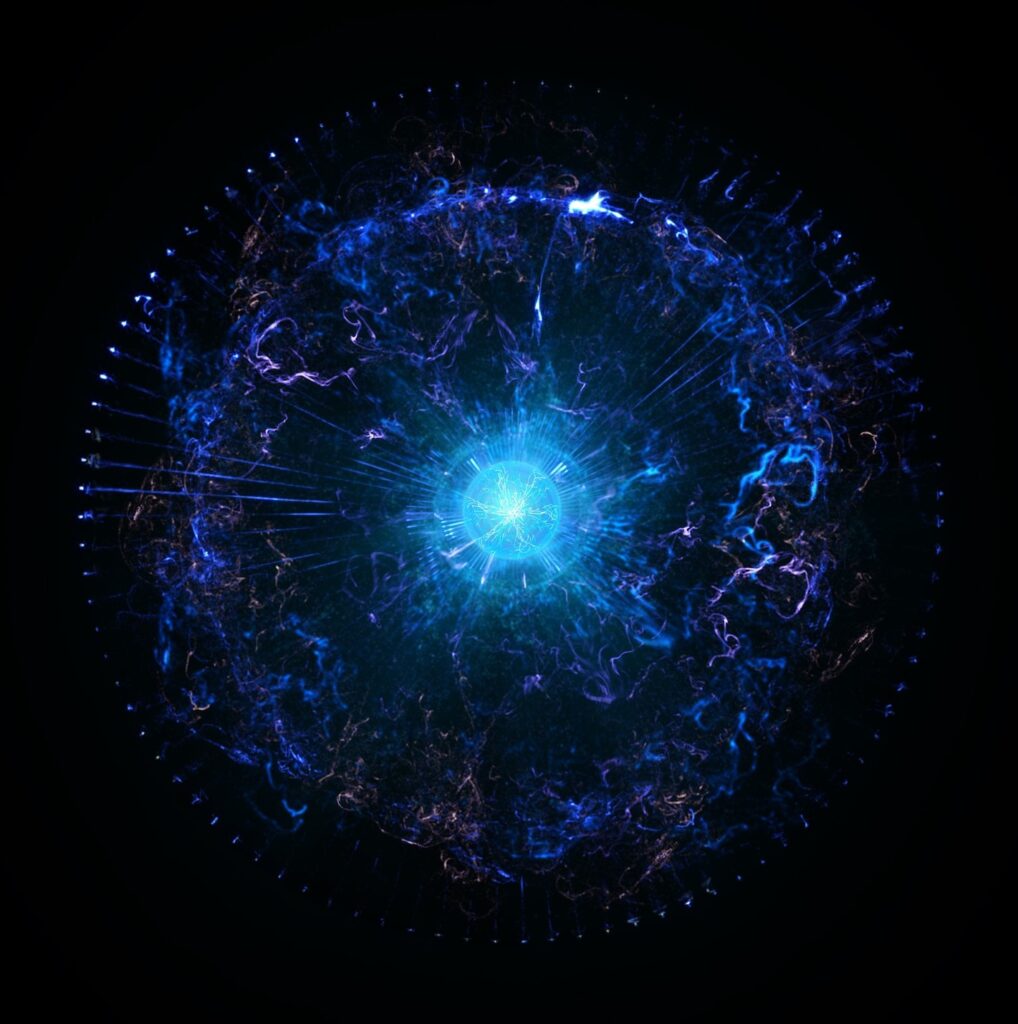
Funding a Fusion Future: From Billionaires to SPACs
The surge in fusion entrepreneurship wouldn’t be possible without a matching surge in capital willing to underwrite high-risk, high-reward technology development. In the U.S., fusion startups have drawn investments from Silicon Valley icons, oil and gas corporations, institutional investors, and even sovereign wealth funds, all betting that fusion could be the ultimate disruptor in the trillion-dollar energy market. The profile of backers is striking. On one end, you have tech visionaries like Sam Altman (who not only led Helion’s funding rounds but at one point committed personally around $375 million) and Jeff Bezos (investing in General Fusion). On the other, big industry players like Chevron and ExxonMobil have dipped their toes, perhaps seeing fusion as a long-term complement or insurance to their portfolios (Chevron in TAE, and reportedly Exxon in Commonwealth Fusion’s latest rounds). Bill Gates has been a prominent fusion advocate, backing CFS from the start via Breakthrough Energy and also supporting other fusion-related research. Even software companies and data center operators are showing interest – Microsoft’s willingness to sign a fusion PPA with Helion is a prime example of a customer stepping up early, essentially providing a guaranteed market if the technology arrives. That Microsoft deal also implicitly encouraged investors that there could be enormous commercial demand for whoever gets fusion right first.
By mid-2023, the Fusion Industry Association reported over $6.2 billion total private investment across the sector, and by late 2024 that figure surpassed $7 billion. Commonwealth Fusion’s $1.8B round in 2021 still stands as the largest single haul, but Helion’s and TAE’s hundreds-of-millions rounds, and multiple smaller companies raising $50M+ rounds, have quickly added up. Importantly, much of this capital is “smart money” that comes with technical vetting. For instance, CFS’s series B included not just VCs but strategic partners like Eni, the Italian energy giant, which has expertise in large energy projects and sits on CFS’s board, lending engineering help as well. Similarly, when Nucor (the steel company) invested in Helion, it signaled interest in how fusion might supply heavy industry with clean energy in the future. This mix of investors suggests a convergence of Silicon Valley-style innovation with industrial know-how.
Public markets have so far remained mostly on the sidelines – no fusion startup has yet had a traditional IPO. During the SPAC boom of 2020–2021, there was speculation that some fusion players might go public via blank-check companies. In fact, U.K.-based Tokamak Energy entered talks with a SPAC (Magnum Opus) to list on the NYSE, but that deal was not completed and the SPAC ultimately shut down without a merger. The general market downturn and the reality that fusion firms still have years of development ahead likely made public listing less attractive for now. Instead, fusion companies have found ample private funding at increasing valuations, allowing them to stay private longer. Commonwealth Fusion, Helion, and others may eventually tap public markets or large project finance for the billions it will cost to build actual plants. We are already seeing early moves in that direction: CFS’s partnership with the Virginia state government and Dominion Energy to site the ARC plant is a prelude to seeking broader financing and perhaps government support for construction. Similarly, Type One Energy’s collaboration with TVA could ease its access to federal infrastructure funding or loan guarantees when it comes time to build Infinity Two.
Government funding itself is also playing a catalytic role. In the U.S., the Department of Energy launched a Milestone-Based Fusion Development Program in 2022, which awarded an initial $46 million in cost-share grants to eight private fusion companies. Notably, Type One Energy was among the awardees, along with Commonwealth Fusion Systems, Tokamak Energy, Xcimer, Zap Energy, and others. These milestone awards are structured so that companies receive federal payments only upon hitting agreed technical targets – an approach modeled after NASA’s partnerships with SpaceX and others for commercial spaceflight. By design, it stretches limited public funds while incentivizing progress and giving the companies an official stamp of credibility when they meet milestones. In fact, DOE noted that after the program’s announcement, the awardee companies collectively raised over $350 million in new private capital, roughly an order of magnitude more than the government money, demonstrating the leverage of public support. This public-private synergy is likely to grow. At a White House fusion summit and subsequent congressional hearings, U.S. officials have made clear they view fusion as a critical part of the clean energy transition and are keen to maintain U.S. leadership in the field. Legislation has been introduced to increase fusion R&D funding, create regulatory frameworks (more on that next), and even to establish fusion-energy development facilities in partnership with industry.
All this is to say, while fusion was once the realm of pure science funded by governments, today it’s an emerging industry drawing talent and money from many directions. The optimism is tempered by realism among experts – as one fusion scientist quipped, “It’s great progress, but Mother Nature isn’t easily fooled. This is still one of the hardest science-engineering problems ever attempted.” Yet, for perhaps the first time in history, fusion companies have to worry about things like hiring hundreds of engineers, securing supply chains for exotic materials, scaling up component manufacturing, and meeting customer delivery dates. Those are good problems to have, because they indicate the field has moved beyond conjecture into execution.
The Last Mile Challenges: Regulation and Grid Integration
As fusion projects shift from lab demos toward full-scale power plants, they face new kinds of challenges that the industry has never had to grapple with before: How do you license a fusion reactor for operation? How do you ensure it can be connected safely to the electric grid and run reliably? How will maintenance and fueling work in a power plant setting? These practical questions are now front and center.
One positive development on the regulatory front came in April 2023, when the U.S. Nuclear Regulatory Commission (NRC) made a landmark decision to regulate fusion differently from fission reactors. In a unanimous vote, the NRC commissioners agreed that fusion energy devices would be overseen under the framework used for particle accelerators and “byproduct” nuclear materials (10 CFR Part 30), rather than the much heavier fission reactor regulations (10 CFR 50/52). This was exactly what the fusion industry had been lobbying for. It means that a fusion plant will not need the same kind of full nuclear power plant license as a conventional nuclear reactor, recognizing that fusion can’t meltdown or run away like fission. Instead, regulation will focus on the radioactive materials a fusion plant does handle – primarily tritium fuel and any activated materials from neutron bombardment – similarly to how particle accelerator facilities or industrial irradiation machines are regulated. The NRC is now working on a limited rulemaking to adapt the byproduct material rules to fusion specifics and to guide U.S. states (many of which have agreement authority to regulate nuclear materials) to be ready for commercial fusion facilities. The bottom line: in the U.S., getting a fusion pilot plant licensed should be a more straightforward, agile process than licensing a new nuclear fission plant. This regulatory certainty is crucial for companies like Type One and CFS as they plan construction – it reduces one layer of risk for investors and partners. It’s worth noting that other countries are moving similarly; the U.K., for instance, has indicated it will not treat fusion as high-hazard nuclear in regulatory terms, and Japan and others are reviewing policies as well.
That said, “not regulated like fission” doesn’t mean “unregulated.” Fusion plants will still have to meet rigorous safety standards for containing tritium (a radioactive form of hydrogen) and shielding people and the environment from neutrons and any activated components. A D-T fusion reactor produces 14 MeV neutrons that can make the reactor structure mildly radioactive over time (from neutron activation of metals) and can generate secondary waste like irradiated cooling water or components. In the words of former White House science advisor John Holdren, while fusion is cleaner than fission, “the first generation plants will have a lot of tritium, and neutrons will create radioactivity in the structural materials… We should not mislead the public that this is the holy grail of absolutely clean, dirt cheap energy. Ain’t going to happen”. In other words, fusion will still need careful engineering for safety – just that the nature of the hazards (tritium and neutron-activated materials) is more akin to industrial radiation facilities than nuclear reactors. Type One Energy’s design, for example, explicitly plans for a tritium breeding blanket with proper shielding, and their partnership with Oak Ridge (a lab with deep nuclear expertise) likely helps in devising safe fuel cycle systems. Fusion developers will have to prove to regulators that they can handle tritium without significant releases (tritium is radioactive and can form radioactive water if leaked) and that their reactor’s neutron emissions won’t pose a danger outside the plant boundary. Given the smaller footprint of many private fusion designs, these challenges seem manageable with current technology (tritium handling systems exist from heavy water reactors and ITER, and materials like steels and tungsten can be used to absorb neutrons).
When it comes to grid integration, fusion plants will behave in some ways like traditional power plants and in other ways like novel sources. A fusion pilot plant like Infinity Two or ARC will deliver on the order of a few hundred megawatts, which is well within the scale that today’s grid can handle as a single generator. It would connect to the grid via a transformer yard much like a comparable gas or nuclear plant. Utilities like TVA and Dominion are already considering the integration: for instance, Dominion will provide technical expertise to ensure CFS’s ARC plant can sync with Virginia’s grid needs. One integration consideration is power output profile. Stellarator and tokamak fusion plants are expected to run fairly continuously at a set power level – effectively providing steady baseload power, or potentially load-following if they can be throttled. In that sense, they’d function a lot like current nuclear plants or large combined-cycle gas plants. Grid operators would welcome the inertia and stability provided by a steady fusion generator, especially as more intermittent renewables come online. The flip side is that if fusion plants run best at steady state, they may be less adept at rapid ramping to chase demand peaks (at least in early years), meaning they’d be complemented by flexible resources.
Helion’s concept introduces an interesting twist: because it’s pulsed and aiming to directly produce electricity as alternating current, the reactor’s pulse frequency could be tuned to grid frequency (60 Hz in the U.S.). Kirtley noted that while their first commercial systems might pulse a few times per second, they envision eventually achieving 60 pulses per second so the power output is effectively smooth AC at 60 Hz. That’s a unique form of integration – essentially designing the fusion pulse rate to match the grid’s cycle so that no complex power electronics or conversion is needed. In the nearer term, Helion will likely use converters/inverters to turn its lower-frequency pulses into usable power. For steady-state machines (tokamaks/stellarators), the output will be thermal energy that drives a turbine-generator, so it will naturally produce AC electricity via the generator, just like any thermal plant. Some fusion startups are exploring advanced heat cycles – for example, using supercritical CO₂ turbines or other high-efficiency generators – which could raise integration questions, but largely it’s standard power engineering.
One challenge to integration and operation that fusion shares with other power plants is maintenance downtime. Replacing components that face intense fusion conditions (like inner wall panels, divertor targets, etc.) could be time-consuming. If a plant has to shut down for months to swap out parts, that lowers its capacity factor and complicates grid planning. Type One seems acutely aware of this; as noted, they’ve “architected a maintenance solution” for good capacity factor – perhaps designing modular internal components that can be changed relatively quickly by robotics or through ports. Still, first-of-a-kind fusion plants will need to prove they can reach, say, 70-80% uptime or better to be economically compelling for utilities. Every fusion design has to wrestle with materials that can withstand the neutron bombardment and heat flux for long periods. There’s a lot of ongoing fusion materials research (like special steel alloys and composites) to extend component lifetimes. Regulators and grid operators will want evidence that these plants can run reliably and won’t trip offline frequently. The early pilots will likely operate at less than full capacity initially as they test and learn – similar to how the first fission reactors ramped up slowly. Grid integration in those cases might involve operating in a “test mode” providing smaller amounts of power at first.
A final integration aspect is public and environmental review. Siting a fusion plant will still require environmental impact assessments, even if the nuclear risk profile is low. The public will want assurances that, for example, if there’s an accident, it won’t spread radiation. Fusion plants don’t have a risk of runaway reactions, so the worst-case accident scenario is likely something like a large tritium release or a magnet quench (loss of superconducting magnets) that damages the machine. Those are local hazards, not wide-reaching. Nevertheless, companies like Type One and CFS are going through environmental reviews as part of their planning. So far, fusion has enjoyed broad bipartisan support and relatively little public opposition (especially compared to fission), but real projects will test that. In Washington state, Helion’s future plant for Microsoft will presumably go through state siting processes; in Tennessee, TVA’s involvement means established procedures for public engagement are in place (TVA has experience adding generation with community input). If fusion plants repurpose old coal plant sites, as TVA is considering, that could ease the way since those sites are already energy-industrial locations, often with communities that are eager for new investment after coal closures. It’s telling that both Type One and Commonwealth are planning to use existing power plant sites – a pragmatic choice to smooth grid interconnection and local acceptance.
Energy Security and Climate: What Fusion Means for the U.S.
If ventures like Type One Energy’s stellarator and its competitors succeed, the implications are hard to overstate. For the United States, commercial fusion power would represent a major leap in energy security, economic competitiveness, and climate leadership.
From an energy security standpoint, fusion offers a domestic, virtually inexhaustible resource. The fuels – deuterium and lithium (for breeding tritium) – are abundant or readily obtainable. Deuterium is found in seawater (every cubic meter of seawater contains about 33 grams of deuterium), and lithium is mined in several countries including the U.S. (and can be sourced from brines, geothermal fluids, even seawater in the future). Unlike oil or gas, no nation can embargo deuterium, and lithium supply, while something to manage, is far more geographically distributed than, say, uranium enrichment for fission. A fusion-powered America would need no fuel imports to run its reactors; it could theoretically supply its own fusion fuel for millennia. That could reduce reliance on foreign energy and cushion the economy from global commodity shocks. It also changes the geopolitical energy map – countries with strong fusion technology might effectively leapfrog those with fossil fuel reserves.
Fusion plants would also bolster grid resilience. Because they don’t require continuous fuel shipments (like coal or gas plants do) and can be placed almost anywhere (not needing specific geologic repositories like geothermal or constraints like hydro), fusion could be deployed in diverse regions to provide always-on power. That complements renewables beautifully: as the U.S. grid incorporates more solar and wind, having a carbon-free “firm” generation source helps fill gaps when the sun isn’t shining or wind isn’t blowing. Energy experts often talk about the need for firm, dispatchable clean power in a net-zero grid by 2050 – fusion is one of the candidates for that role (alongside long-duration storage, geothermal, advanced fission, etc.). If Infinity Two or ARC come online by the 2030s, they might initially just prove the concept. But by the 2040s, if replicated, fusion plants could take over the role that coal plants once played: providing continuous, adjustable output to stabilize the grid and meet the base demand, but without the emissions or fuel supply vulnerabilities.
For U.S. economic and technological leadership, being at the forefront of fusion can have far-reaching benefits. It’s not just about energy; it’s an entire advanced industry – high-tech manufacturing for superconducting magnets, robotics for reactor maintenance, new materials science, power electronics, and more. The know-how gained from building the first fusion plants will likely spill over into other industries. We saw a parallel with the space industry: SpaceX’s innovations didn’t just get rockets flying; they advanced materials, computing, and manufacturing techniques that have cross-pollination effects. Fusion could spawn similar clusters of innovation. It could also become a major export industry. If U.S. companies like Type One, CFS, Helion, or others develop viable reactors, the global demand for clean energy is so vast that exporting fusion power systems or licensing the technology could be a huge economic boon. Countries aiming for deep decarbonization – from Japan to India – might prefer buying a proven fusion reactor design from the U.S. rather than reinventing the wheel, much as nations bought nuclear reactors or gas turbines from those who pioneered them. In essence, leading in fusion could ensure the U.S. is an energy exporter not of fuel, but of technology and equipment, in the latter half of the 21st century.
On the climate front, fusion directly addresses the need for massive-scale, zero-carbon energy. Solar and wind are ramping up impressively and will carry much of the load through 2030. But as we push toward 100% clean grids and electrify transportation, heating, and industry, the sheer volume of clean electricity needed becomes enormous. Some analyses indicate global electricity generation may need to roughly triple by 2050 to cover all this – and all of it must be carbon-free. That is an enormous challenge even if we deploy renewables at breakneck speed. Fusion, if available in time, could relieve some of that pressure. It could, for instance, provide continuous power to produce green hydrogen at scale (for use in heavy transportation or steelmaking), or power energy-intensive industries that are hard to run on intermittent sources. It could also complement seasonal cycles – perhaps running steady in winter when solar is lower, etc., though that’s more of a grid management detail. The key is that fusion doesn’t emit CO₂ or air pollutants; its only byproducts in D-T are helium (harmless) and some neutron-activated materials that remain on site.
One often-cited criticism is that fusion might come “too late” to help with climate change, given urgent 2030 targets. It’s true that fusion will not significantly cut emissions in this critical decade – it simply won’t be ready for large deployment that fast. But fusion’s real contribution is more toward the 2040s and beyond, aligning with mid-century climate goals. By 2050, the world may need to not only reach net-zero emissions but possibly net-negative, all while supporting a larger population and higher energy demand. Fusion could be a game-changer in that era: a clean energy source that’s effectively limitless, allowing continued economic growth without carbon. Jennifer Granholm, the U.S. Energy Secretary, highlighted in 2022 that “the landscape around fusion research and development is shifting” and that to meet future demand, the strategy must shift too, integrating fusion alongside renewables and other tools. In sum, fusion is part of the long-term climate solution toolbox, ensuring that we don’t hit a wall in emissions reductions in the 2035–2045 period when the easier wins from efficiency and current renewables have been exhausted.
There’s also a national security angle: being the first to harness fusion energy at scale could confer strategic advantages. It’s a prestige project on one hand – demonstrating scientific leadership – but also a practical one. If the U.S. can build reliable fusion power plants, its military and critical infrastructure could eventually be powered by fusion, making them more self-sufficient and resilient. And consider a world where perhaps not oil, but fusion technology, is what countries vie over. If the U.S. is the leader, it can set norms and practices (for safety, nonproliferation of any neutron source concerns, etc.). This is analogous to how the U.S. led with GPS satellites or the internet – establishing early dominance can define standards globally. Fusion doesn’t pose direct weapons proliferation risks in the way fission does, but the neutrons from D-T could be misused to breed fissile materials if someone tried very hard. Having leading expertise allows the U.S. to guide international agreements on what commercial fusion devices should look like and how to prevent any misuse.
For the average person, the advent of fusion could eventually mean plentiful electricity with less volatility in price. Fusion fuel costs are negligible in the long run (the “fuel” is basically water and lithium, extremely cheap per unit of energy). Almost all the cost is in building the plant. So if those capital costs can be brought down with economies of scale, fusion plants might produce power that is not only clean but also very cheap per kWh. That could boost economic productivity and lower energy bills, albeit that’s likely decades out. In the nearer term, just proving that a fusion plant can operate will be a major psychological turning point – showing that humankind can create a small sun on Earth to light our cities.
Of course, caution and realism are warranted. As Dr. John Holdren warned in 2024, despite great progress, “we will not see economically competitive fusion on the power grid before 2050” in his view, and he’d bet on that timeline. Many in the traditional fusion research community share the sentiment that the challenges from demo to commercial rollout are immense. First-of-a-kind energy projects often face cost overruns and delays (just look at recent nuclear reactor builds or even some large renewable projects). Fusion plants will be complex and require precision – a lot can go wrong in construction or initial operation. It’s conceivable that Infinity Two or ARC, if built, might encounter setbacks or take longer to optimize. And the economics are not yet proven; a machine that works scientifically is not automatically a machine that is competitive in the electricity market. Fusion must ultimately compete with increasingly cheap renewables plus storage, as well as with other clean firm sources (advanced fission, geothermal). If it can’t get costs down, it might remain a niche. But the bet many are making is that with technology learning curves and enough deployment, fusion can achieve cost parity and then undercut other sources because of its fuel advantages and high power density.
For now, in 2025, it’s an exhilarating moment in fusion development. Type One Energy’s stellarator announcement adds a new flavor to the mix – reminding everyone that the fusion race isn’t one-dimensional. We have donuts and pretzels, giant magnets and colliding plasma bullets, lasers and piston-compression, all in the fray. It’s as if after decades of one slow-moving plot, the fusion story has split into multiple thrillers running in parallel. The U.S. finds itself with a strong hand: home to many of the top private fusion ventures and enjoying growing public support for fusion R&D. The next decade will determine if one or more of these approaches can break through the remaining barriers. If they do, the payoff is not just a scientific triumph but a transformative new industry.
As Christofer Mowry of Type One said, the goal now is “reliable electrical generation for the power grid” – to make fusion something that keeps the lights on, day in and day out. Achieving that will mark a historic inflection point: fusion energy moving from the realm of experiment and promise into the fabric of daily life. It’s the kind of development that could shape the 21st century as profoundly as oil shaped the 20th. We are not there yet. But with initiatives like Infinity Two, the first glimmers of the fusion era are coming into view – not in a starry far-off future, but within the working plans of utilities and engineers today. The stars may soon be coming down to Earth, one power plant at a time.